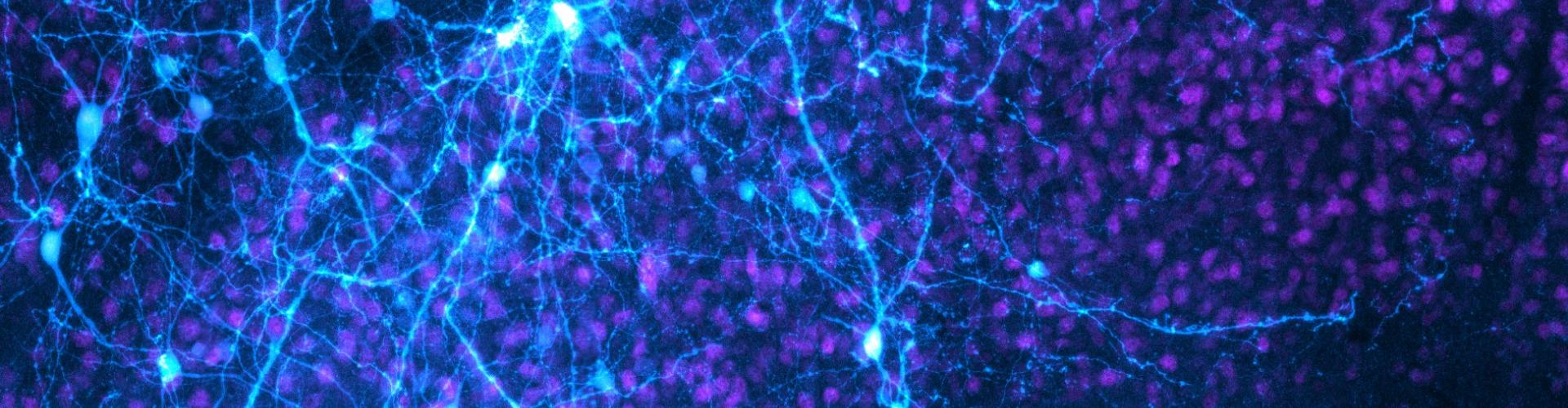
Masters of Escape: Investigating the neural circuits for survival
What would you do if someone (or something) came at you with the intention of eating you? Would you try to outwit it by blending in with the scenery? Would you make use of your ninja skills and kick it in the face before running away? Or would you keep it cool and wait until this something realised it doesn’t have the guts to digest you? Regardless of your strategy, chances are an animal out there employs it better.
Hiding in plain sight
Certain species of octopus have mastered the art of keeping it cool even if a hungry shark is swimming nearby. As a first line of defence, they may go for the strategy of casually grabbing a few shells from the sea floor, covering themselves, and waiting in disguise until the not-so-friendly shark decides to continue his foraging somewhere else. Although being clever may work for a while, even the coolest of the octopuses has a limit. If nudged too much by the hungry shark, the octopus will move to stage II of the escape plan: shedding its armour of shells and darting away while the hungry shark searches for a dinner that is no longer there.
A partnership for posterity
The Hawaiian bobtail squid is no octopus, but it struck a very fine deal with the glowing bacteria Vibrio fischeri in order to secure its own future. In exchange for food and a place to live, the bobtail squid harnesses the bioluminescence of the bacteria to generate a blue glow that hides its silhouette and allows it to blend with the surroundings. An exquisite partnership to minimise unwanted encounters with predators.
Jump, kick, run!
Playing it cool and hiring little minions to hide you is all very well, but sometimes a disguise is not enough. For nocturnal desert rodents like the kangaroo rat, it is not always possible to avoid being detected by unexpected predators lurking in the dark. When faced with an attack by a snake, the kangaroo rat smoothly jumps out of the snake’s trajectory, turns mid-air, and hits the attacker across the face with its tail. That mid-air pivot is not just for defence: it also allows the rat to orient in such a way that it hits the ground facing an escape route.
It is never too late
Not all animals are great at escaping. The ostracod is a tiny crustacean that lives underwater. It’s among the smallest animals in the pond, and not the best swimmer. And when you are the smallest and you can’t swim very well, sooner or later you will end up in someone else’s digestive tract. Knowing one’s own limitations can be an asset in these situations, and although ostracods may not be great at escaping before they’re eaten, there’s no reason they can’t escape after they’ve been eaten. Their secret weapon is ejecting luciferin and luciferase, two compounds that together trigger a reaction that produces carbon dioxide, oxyluciferin, and blue light—bioluminescence. The light this reaction generates is so bright it can attract larger predators, making the original snacker quickly realise their mistake and release the ostracod.
A somewhat similar yet less beautiful strategy is the one adopted by the bombardier beetle after being eaten by a toad. Instead of luciferin, the beetle ejects a hot chemical spray that makes the predator vomit. Not elegant, but an escape nonetheless.
Unravelling the neural circuits for escape behaviour
Escaping from a predator is one of the most adaptive elements within the repertoire of instinctive behaviours animals can execute in their struggle for survival. It’s also one of those behaviours under critical evolutionary pressure where a few milliseconds of delay between selection and implementation can lead to a fatal outcome. We know it’s hard-wired into the neural pathways of a species, but neuroscientists remain unsure of exactly how it works. At the Sainsbury Wellcome Centre we’re trying to figure it out.
Using mice as a model organism and an experimental paradigm where an expanding shadow mimics the attack of a bird of prey, the Branco lab has identified two midbrain areas that are key for estimating the level of threat in a given situation and triggering the escape response if the threat level exceeds a critical value. Knowing where the decision to escape is implemented has paved the way for further research and opened new lines of investigation.
Although different species can adopt different strategies to escape from predators, the decision of when to execute the preferred strategy remains at the core of an individual’s survival. We still don’t know how the critical level of threat above which escape is triggered is modulated by other factors such as stress, hunger, and previous experience. And once the decision has been made, how does the escapee reach safety? How does an animal keep track of where their shelter is at all times so that they can take the optimal path to shelter? How and where in the brain is this path updated if an unexpected obstacle appears on the way?
Knowing the answers to these questions will allow us to better understand how the brain constantly updates its model of the world to guide complex behaviours and maximise an individual’s chances of survival. In the long term, this research may also help us understand how severe anxiety or post-traumatic stress disorder change how these neural circuits work, turning situations that should be harmless or safe into being perceived as threatening, as well as help us develop strategies to counteract the negative effects of such debilitating conditions.
For more on the Branco Lab’s work on escape, please see “Cognitive Control of Escape Behaviour”, “Rapid Spatial Learning Controls Instinctive Defensive Behavior in Mice”, and “A Synaptic Threshold Mechanism for Computing Escape Decisions”, or go to the publications page on the lab website.