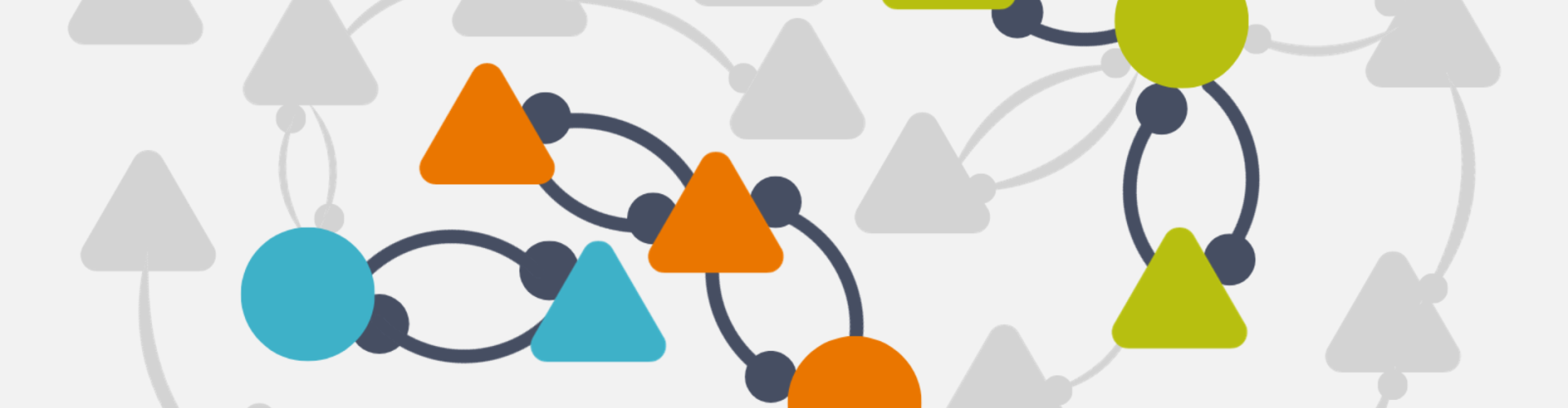
Revealing the hidden precision of inhibitory circuits
By April Cashin-Garbutt
Just like computers are characterised by their hardware, neural circuits in the brain are defined by their wiring. The synaptic organisation determines the function of neural circuits. While the connections of excitatory and inhibitory neurons were previously characterised, a new study has revealed the hidden precision of the synaptic strength of inhibitory circuits in the neocortex.
“People often think of excitatory neurons as doing the bulk of the interesting computations in the brain, whereas inhibitory neurons are thought to coordinate the activity of excitatory cells. We know from previous research that the connectivity of excitatory cells is very specific, whereas inhibitory neurons were thought to have very broad and non-specific connections,” explained Petr Znamenskiy, Group Leader at the Francis Crick Institute and former postdoctoral researcher in the Mrsic-Flogel Lab at the Sainsbury Wellcome Centre.
In a new paper published in Neuron, Znamenskiy, and colleagues at the Sainsbury Wellcome Centre at UCL and Biozentrum at University of Basel, looked more closely at the organisation of inhibitory neurons, specifically focusing on their synaptic strength. Their research revealed unexpected precision of the synaptic strength of paravalbumin-positive (PV+) inhibitory neurons in the mouse visual cortex. This observation could help explain other experimental findings.
Synaptic connectivity and synaptic strength
Synaptic connectivity, or how neurons are connected to each other, plays a key role in determining the function of neural circuits. Almost equally important is synaptic strength. Neural connections range from strong to weak, and the strength of connections can differ by around two orders of magnitude.
“A connection that is a hundred times stronger than another connection is going to have a much bigger impact on the function of that circuit. That’s why looking at connection strength is important. And so, we looked at the organisation of both excitatory inputs from excitatory cells onto inhibitory cells and the strength of inhibitory connections from inhibitory cells onto excitatory cells,” explained Znamenskiy.
The team focused on a particular population of inhibitory cells called PV+ inhibitory neurons, which are one of the major populations of inhibitory neurons in the cortex. PV+ neurons are thought to be one of the key sources of inhibition in the cortex.
Znamenskiy and colleagues presented mice with visual stimuli on a screen and used calcium imaging in vivo to look at their visual responses. Then they prepared brain slices in vitro to electrically access individual neurons to test for the presence of connections between them and measure the strength of the connections.
Revealing unexpected precision
The team found that the organisation of the synaptic strength of the PV+ inhibitory neurons was unexpectedly specific. The inhibitory cells received strong excitation from a subset of nearby excitatory cells and strongly reciprocally inhibited those cells. Furthermore, they found that the cells with strong connections shared similar visual responses.
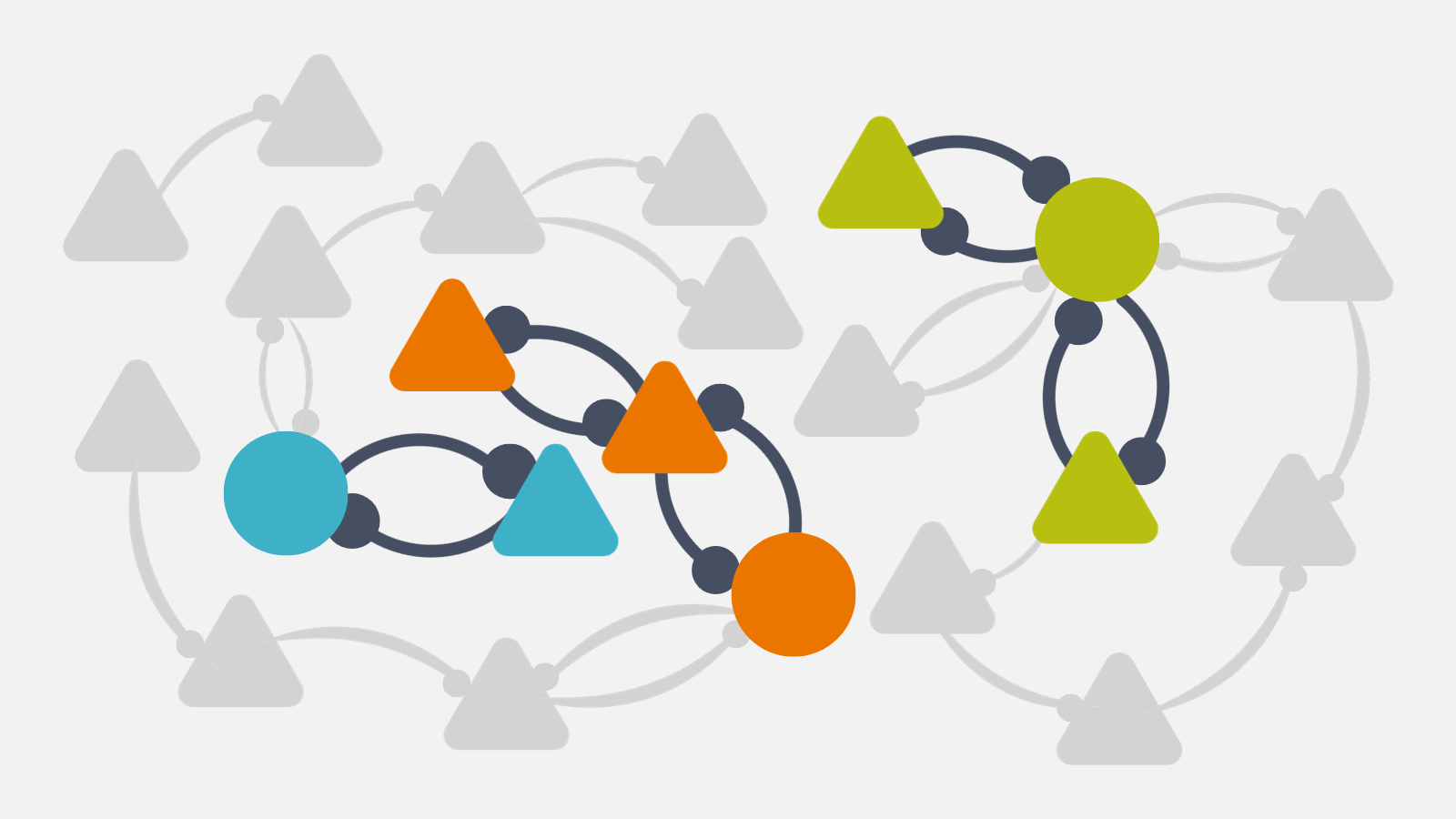
“When we looked in vivo at the properties of the neurons, the ones that were strongly wired had similar patterns of activity and similar visual responses, but the ones that were weakly connected did not have similar responses,” explained Znamenskiy.
In addition, the team were able to reproduce the previous finding, that the presence or absence of connectivity is not specific, thereby explaining why previous research that only looked at the presence or absence of connections concluded that the inhibitory neurons have broad, non-specific connections.
Exploring possibilities with network simulations
To further explore the role of the wiring pattern on the function of the neural network, Znamenskiy et al. used simulations to manipulate the wiring rules and see how this impacted the behaviour of the simulated circuit.
The simulation showed that when the excitatory connections are made more precise, the network eventually becomes unstable. This leads to runaway excitation, where network activity persists in the absence of external input.
For example, if you present a visual stimulus, the cells continue firing even when the stimulus goes away. This is because the network consists of strongly, recurrently connected subpopulations of neurons all tuned to the same stimulus. And so, once the excitatory cells start firing, they continue to sustain themselves and don’t shut down.
Next the team tried changing the rules of inhibitory connections at the same time, by reassigning the strength of connections between different neurons. When they changed the organisation to make inhibition more specific, they found the network returned to stability.
“These simulations show that there is a trade-off between specificity of excitatory and inhibitory connectivity that together determines the dynamics of the network,” explained Znamenskiy.
Building a more complete picture across cell types
These findings overturn a decade-long idea that inhibitory neurons provide blanket normalising inhibition that reflects the overall activity in the local population. Znamenskiy et al. show that for PV+ inhibitory neurons this is not the case.
“We found that PV+ neurons are not providing uniform inhibition. Instead, each one is integrated into specific excitatory ensembles. This organisation could be important for maintaining the stability of the network, which is vital because unstable networks can lead to seizures,” explained Znamenskiy.
Now that the team have determined the connectivity rules for PV+ inhibitory neurons, they want to explore other cell types in the visual cortex. The aim is to build a more complete picture of how connectivity rules differ across cell type diversity. In total there are 14 major cell types in the visual cortex – nine excitatory and five inhibitory. This 14x14 matrix of potentially different connectivity rules together make up the neocortical circuit wiring.
At the current speed of data collection, it would take scientists around 200 years to build a complete picture across all cell types. In Znamenskiy’s lab at the Francis Crick Institute, the team are developing new methods to tackle this question systematically.
“One of the reasons we think evolution has come up with so many different cell types in the cortex is that it makes it possible for them to follow different wiring rules. This allows evolution to shape the computations that cortical circuits perform. That’s why we are excited to understand how other cortical cell types differ in their wiring organisation,” concluded Znamenskiy.
Find out more
- Read the full paper in Neuron, ‘Functional specificity of recurrent inhibition in visual cortex’ DOI: 10.1016/j.neuron.2023.12.013 10.1016/j.neuron.2023.12.013
- Learn more about research in the Znamenskiy Lab and Mrsic-Flogel Lab