Abstract
Hiroki R. Ueda1,2,3
1. Systems Pharmacology, Graduate School of Medicine, University of Tokyo, Tokyo, Japan
2. Laboratory for Synthetic Biology, Center for Biosystems Dynamics Research, RIKEN, Japan
3. Department of Systems Biology, Institute of Life Science, Kurume University, Japan
In this lecture, I will discuss recent advancements in understanding the role of calcium and calcium-dependent phosphorylation in sleep homeostasis. Our research took a significant turn at the international conference "Sleep 2012" in Boston, where we came up with a new hypothesis: sleep homeostasis may not rely solely on "sleep substances," but could be better explained by mechanisms that account for the history of "wakefulness substances," such as calcium. This shift led us to focus our research on calcium as a potential key factor in regulating sleep homeostasis.
Building on Dr. Setsuro Ebashi's classical discovery of calcium as a signaling molecule, we explored the hypothesis that calcium, while stimulating neurons, might also contribute to sleep promotion. Testing this hypothesis required the development of "next-generation genetics," which can be defined as the genetics that do not involve traditional breeding. We developed the "Triple-CRISPR method" in 2016, which enabled us to generate knockout mice with over 95% efficiency in a single generation1. Using this method, we created 25 knockout mouse lines targeting calcium channels and pumps. Our findings demonstrated that calcium plays a role in promoting sleep2, suggesting that it may indirectly function as a brake in regulating neuronal excitability.
We also developed a new tissue-clearing and whole-brain cell profiling technique, CUBIC3,4. This technique allowed us to demonstrate that calcium can suppress neuronal excitability, providing a new perspective on its role in sleep regulation2. We also identified the role of calcium-dependent kinases, particularly CaMKIIα and CaMKIIβ, that integrate calcium history to promote sleep. Further analysis revealed three groups of phosphorylation sites in CaMKII that are critical for 1) sleep induction, 2) sleep maintenance, and 3) sleep cancellation, respectively5. Further reverse genetic screening also revealed a sleep-inhibiting kinase, PKA, and sleep-promoting phosphatases, PP1 and calcineurin, which modulate both sleep quality and quantity6.
Our research also led to the proposal of the WISE (Wake Inhibition Sleep Enhancement) mechanism7 for the role of sleep, which offers an alternative, rather than an exclusive, perspective to the widely accepted SHY (Synaptic Homeostasis) hypothesis. The WISE mechanism suggests that periods of quiet wakefulness generally suppress synaptic connections, while deep sleep serves to strengthen them. According to this model, molecules associated with long-term depression (LTD) during prolonged quiet wakefulness promote deep sleep. This prediction was supported by our recent discovery of calcineurin's role in promoting sleep6.
The WISE mechanism also provides new insights into why chronic sleep deprivation (prolonged wakefulness) can lead to depression, as it is associated with decreased synaptic AMPA receptor expression. Additionally, it helps explain why rapid-acting antidepressants universally increase delta power during sleep. This hypothesis may also clarify phenomena that existing theories struggle to address, such as the increased delta power observed during the recovery phase after hibernation, which is associated with decreased synaptic strength.
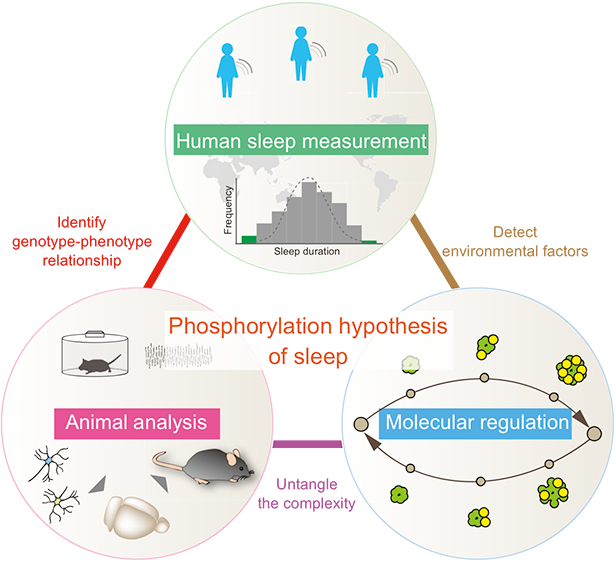
References
1. Sunagawa, G.A., Sumiyama, K., Ukai-Tadenuma, M., Perrin, D., Fujishima, H., Ukai, H., Nishimura, O., Shi, S., Ohno, R.I., Narumi, R., et al. (2016). Mammalian Reverse Genetics without Crossing Reveals Nr3a as a Short-Sleeper Gene. Cell Rep 14, 662-677. 10.1016/j.celrep.2015.12.052.
2. Tatsuki, F., Sunagawa, G.A., Shi, S., Susaki, E.A., Yukinaga, H., Perrin, D., Sumiyama, K., Ukai-Tadenuma, M., Fujishima, H., Ohno, R., et al. (2016). Involvement of Ca(2+)-Dependent Hyperpolarization in Sleep Duration in Mammals. Neuron 90, 70-85. 10.1016/j.neuron.2016.02.032.
3. Susaki, E.A., Tainaka, K., Perrin, D., Kishino, F., Tawara, T., Watanabe, T.M., Yokoyama, C., Onoe, H., Eguchi, M., Yamaguchi, S., et al. (2014). Whole-brain imaging with single-cell resolution using chemical cocktails and computational analysis. Cell 157, 726-739. 10.1016/j.cell.2014.03.042.
4. Tainaka, K., Kubota, S.I., Suyama, T.Q., Susaki, E.A., Perrin, D., Ukai-Tadenuma, M., Ukai, H., and Ueda, H.R. (2014). Whole-body imaging with single-cell resolution by tissue decolorization. Cell 159, 911-924. 10.1016/j.cell.2014.10.034.
5. Tone, D., Ode, K.L., Zhang, Q., Fujishima, H., Yamada, R.G., Nagashima, Y., Matsumoto, K., Wen, Z., Yoshida, S.Y., Mitani, T.T., et al. (2022). Distinct phosphorylation states of mammalian CaMKIIbeta control the induction and maintenance of sleep. PLoS Biol 20, e3001813. 10.1371/journal.pbio.3001813.
6. Wang, Y., Cao, S., Tone, D., Fujishima, H., Yamada, R.G., Ohno, R.-i., Shi, S., Matsuzawa, K., Kaneko, M., Ukai-Tadenuma, M., et al. (2023). Post-synaptic competition between calcineurin and PKA regulates mammalian sleep-wake cycles. bioRxiv, 2023.2012.2021.572751. 10.1101/2023.12.21.572751.
7. Kinoshita, F.L., Yamada, R.G., Ode, K.L., and Ueda, H.R. (2024). Boundary conditions for synaptic homeodynamics during the sleep-wake cycle. bioRxiv, 2024.2008.2014.607872. 10.1101/2024.08.14.607872.