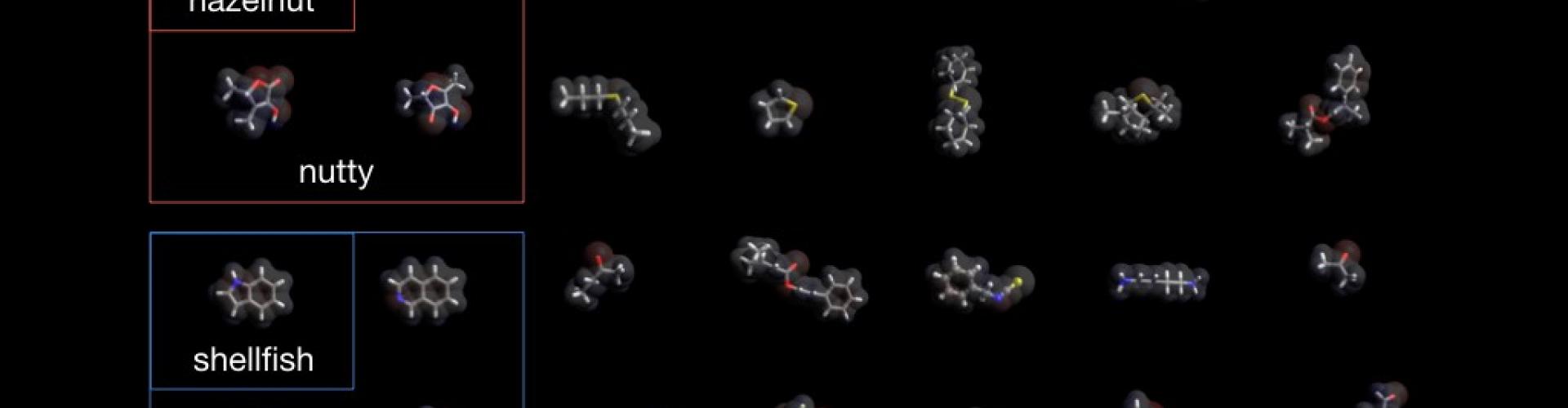
Detecting, discriminating and generalising odours
An interview with Dr Sandeep Robert Datta, conducted by April Cashin-Garbutt, MA (Cantab)
How does the brain allow us to achieve two seemingly competitive tasks: telling odors apart and knowing that different smells are related to each other? While both involve detecting an odour, the former requires the ability to perceive differences, whereas the latter requires a focus on similarities.
In the following interview, Dr Sandeep Robert Datta, from Harvard Medical School Department of Neurobiology, outlines how his lab are characterising the olfactory system to elucidate how animals use their sense of smell to interact with their environment and generate odour-triggered behaviour.
Why do you focus on the olfactory system? What can this particular sensory system teach us about sensory perception?
My main interest is in trying to understand how the brain takes in information about its surroundings and converts that into some sort of meaningful pattern of behaviour. For the vast majority of animals, the main sense that they use to interact with the world is their sense of smell.
Olfaction was the first evolved sense and in mammals the parts of the brain that are devoted to smell are the most evolutionarily ancient. As a consequence, the system that our brain has for detecting smells is almost directly connected to parts of the brain that are involved in emotional memory, like the hippocampus and the amygdala, in a way that other sensory systems are not.
And so, one reason that I’m interested in studying the olfactory system is because it is shallow, it is a fast route into important parts of the brain that let animals adapt to the world.
The other reason why I’m interested in the olfactory system is because it is so intimately connected to evolution. The receptors that detect smells in the world, which are expressed by sensory neurons in your nose, are evolving as fast or faster than any gene family in your genome. Because of the importance of smell, evolution actively sculpts the olfactory system of each species to suit its particular ecological niche and it does so very rapidly. Evolution has also hardwired specific neural circuits into the olfactory system to drive or modulate behaviors in response to important odors, like the scent of food, or the odour of a predator.
I’m interested in the olfactory system in part because it provides a venue to understand how evolution has shaped part of the brain, but also because it is such a flexible system it allows us to see how individual experience over the lifetime of an animal shapes their brain.
Does the close connection of the olfactory system to the hippocampus and the amygdala explain why certain smells can bring back vivid memories for people?
A lot of work has been done in this space and we know from our work and others’ that there are as few as two synapses between your nose and your amygdala. If you compare that to other sensory systems, where there are arguably tens of synapses between a sensory cue and your amygdala, you get a sense of the intimate relationship between your nose and emotion.
A little appreciated fact is that the ongoing input your amygdala gets from your nose is very important for your overall emotional state. People who suddenly lose their sense of smell can often feel rather emotionally adrift, and depression is a common side effect.
Furthermore, a highly validated rodent model for depression in the lab is to disrupt an animal’s sense of smell, and many of the antidepressants that are actually used in the clinic today were in part developed around figuring out how to make an animal who has suddenly lost its sense of smell feel better.
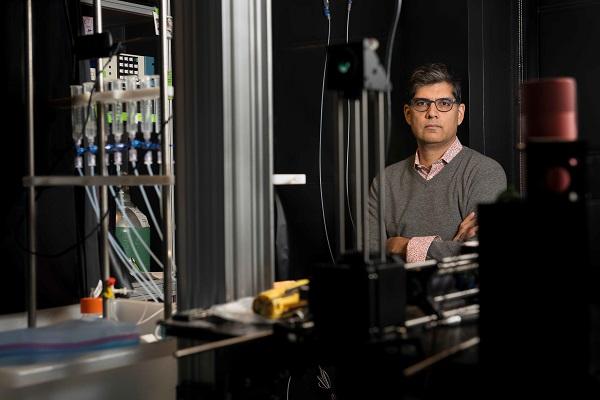
Why does the olfactory system evolve so quickly?
I think it evolves so quickly because chemical information is so prominent in the environment and animals are in a never ending war with their predators to survive. And so, predators have evolved receptors for their prey, and prey have evolved receptors for the predators, and as a consequence, as predator/prey relationships change across evolutionary timescales, the olfactory system changes to match. The particular ecological niche an animal resides in also changes rapidly, and animals have to evolve specific receptors and circuits to manage that. The olfactory system is almost a molecular recorder of an animal’s environmental history.
How does the brain discriminate between different odours?
This is an extremely important problem and it is clear that at some level, the brain tells things apart because your nose can tell things apart.
Odours or smells, are small volatile molecules that come off substances and are detected by receptors in the nose. Different odours will interact with different receptor subtypes and so the degree to which we can tell things apart fundamentally depends on the degree to which the receptors in our nose differentially interact with odours in the world.
What is interesting is that your brain organises information about smells in a way that is largely designed to enhance differences between odours. If you have two odours that are very similar, and activate very similar patterns of receptors in the nose, a lot of what your brain is doing is reconfiguring and reformatting information to allow it to easily separate information about those two odours, which underlies our rather amazing ability to tell smells apart.
How many smells can we tell apart?
This is still very much a matter of open debate. Low estimates are in the tens of thousands of discriminable odours and high estimates say a trillion odorants. I’m certain the truth is somewhere in between.
Regardless of what the number is, it is a spectacular number of things that we’re able to tell apart using our noses. It is clear that the brain has mechanisms that are specifically designed to help us with that task, and to help us tell things apart, even if they’re very closely related. That, of course, explains the ability of perfumers to perform their art, and of winemakers to bring pleasure to the world due to their ability to understand subtle differences between odours.
Are there a lot of genetic differences in terms of what smells people can and can’t distinguish?
We don’t yet fully understand the interaction between genetics and perception in the olfactory system. The genes that make up the receptors for olfaction are some of the fastest evolving genes in the genome, which means that from a practical perspective, your genes for smell are quite polymorphic and different from someone else’s.
We inherit one copy of each receptor gene from our mother and father, and in many cases, those copies themselves are different. There is a lot of functional variation in the olfactory system, and that, at least in part, explains why each of us has a unique sense of smell.
It is difficult to put a number on what extent our sense of smell is common between us. But in my lab, we have recently done some experiments that give us a sense in mice of how similar their odour perception is across individuals.
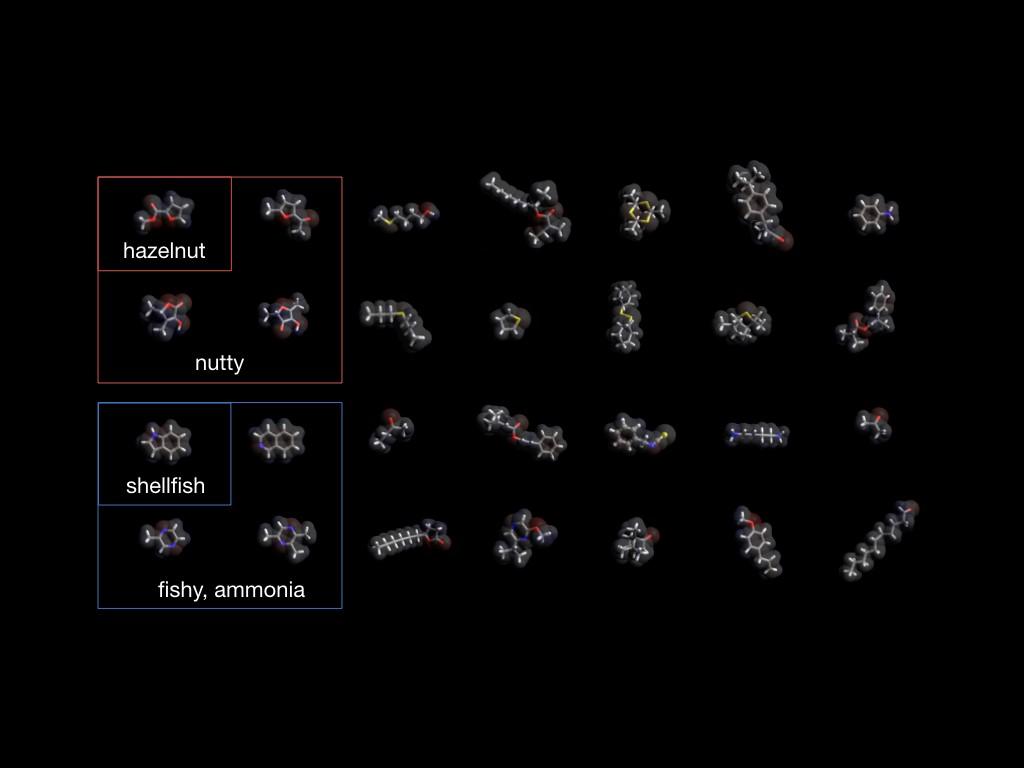
What does it mean to generalise amongst odours and why is this an important ability for the brain?
If I smell a cup of coffee, I know that it is coffee whether or it is an espresso, a cappuccino, a latte, or just plain black coffee. In all cases, I know a key thing, that it is a hot brown liquid that I have to have in the morning otherwise I’ll fall asleep during the day!
As you can imagine, there are many, many circumstances in which animals need to be able to generalise across odours to determine that similar odours are associated with similar kinds of objects in the world, in order to allow them to make appropriate choices.
To some extent, one can argue that every time you smell a cup of coffee, it is slightly different: a different person made it, the beans are different, the water is different, where you drink it is different and yet, somehow there’s something that unifies all of them, perceptually, to us.
Generalisation allows us, without prior experience, to make accurate predictions about the world around us, it is an essential component of perception.
While we’ve had a quite rich understanding of how odours are discriminated by the olfactory system, I think one of the big holes in olfaction is that we don’t fully understand how odour generalisation is supported, particularly by the cortex, the part of the brain that’s responsible for perception.
Recent work in my lab focused on trying to understand how it is that on the one hand, our brains are spectacular at discriminating amongst odorants, but at the same time, they maintain enough information about odour chemistry to allow us to generalise across odorants.
Telling things apart necessarily means separating things and generalising involves grouping things. One of the exciting things that my lab has been working on recently is figuring out how the brain balances these two potentially competing tasks.
What methods do you use to understand the generation of odour-triggered behaviour?
My lab is an interdisciplinary lab, and so we view the problem of trying to understand how odours generate behaviours, from the perspective of: the genes that detect odours; the neural circuits that encode information about odours; the circuits that then decode that information, to translate that information into actions; and the behaviour itself. And so, the toolkits that we use really span all of those questions and disciplines.
We use techniques from molecular genetics to better understand how genes in our genome allow us to sense odours. We use modern tools from systems neurobiology like multiphoton microscopy and in vivo use of head-mounted miniscopes to better understand how patterns of activity might relate to odours in the world. We use modern causal techniques like optogenetics to manipulate neural circuits to get a sense of how odour related decisions might be made.
My lab has developed a brand new method called Motion Sequencing (MoSeq). MoSeq combines 3D machine vision techniques and newly developed types of machine learning to effectively decode mouse body language. Just like human patterns of movement are highly structured allowing us to communicate with one another because of the regularities in the way we move our bodies, we’ve hypothesised that the behaviour of all animals is structured and patterned.
We’ve built new tools that, in an automated way without any human supervision or intervention, allow us to identify the syllables that make up mouse body language and the grammar that structures mouse body language. We’re very excited about this new behavioural tool because I think it provides a real objective view into how odours drive different forms of behaviour.
One very simple example of a thing we can do, is that if you give a mouse, the scent of a fox, or a ferret, or a cat, you look at that mouse and see that, in all cases, the mouse runs away from the scent and hides in the corner. The same goal oriented behaviour is generated by all three of these odours.
However, if you use motion sequencing to analyse the pattern of behaviour, it is clear that the mouse is distinguishing all three of these predators, and generating a predator specific pattern of behaviour that likely reflects evolution’s prior knowledge of these different predators and maybe different ways the mouse can most efficiently escape.
So we’re learning really important new details about how the brain organises behaviour on a moment-from-moment basis, from this analysis of body language.
What is the next piece of the puzzle your research is going to focus on?
My lab has recently combined motion sequencing technology with the ability to record neural activity in the brain. From that work, we’ve been able to effectively predict ongoing patterns of naturalistic behaviour from brain activity, and vice versa. We can predict brain from behaviour and behaviour from the brain.
There’s a particular part of the brain, called the striatum, that seems to be particularly important for specifying the grammar of behaviour, how different components of behaviour are strung together. It is clear that for many innate odour-driven behaviours, fundamentally what they’re doing is causing changes in behavioural grammar to allow animals to generate new patterns of actions to deal with the world.
One thing we’re very interested is in trying to understand how sensory information about smells filters its way into the striatum and changes patterns of activity within the striatum itself – that’s a major area of research in my lab going forward.
About Dr Sandeep Robert Datta
Sandeep Robert Datta obtained a Bachelor of Science degree in Molecular Biochemistry and Biophysics from Yale University in 1993, and obtained an M.D./Ph.D degree from Harvard University in 2004. After working as a postdoctoral fellow at Columbia University with Richard Axel, he joined the Harvard Medical School Department of Neurobiology in 2009.
His lab focuses on understanding how sensory cues — particularly odors — are detected by the nervous system, and how the brain uses information about the presence of salient sensory cues to compose complex patterns of motivated action on a moment-to-moment basis. This work involves studying genes involved in detecting odors, revealing the patterns of neural activity deep in the brain that encode sensory maps of the outside world, exploring motor circuits that transform sensory codes into action, and probing the fundamental statistical structure of behavior itself.
Dr. Datta has published numerous articles on his research in journals including Cell, Science and Nature, is a reviewer and an editor at multiple scientific journals, is an Associate Member of the Broad Institute, and is a Principal Investigator in the Italian Institute of Technology/Harvard Medical School joint program in the neurosciences.
Dr. Datta has received the NIH New Innovator Award, the Burroughs Welcome Career Award in the Medical Sciences, the Alfred P. Sloan Research Fellowship, the Searle Scholars Award, the Vallee Young Investigator Award, the McKnight Endowment Fund Scholar Award and has been named a fellow of the National Academy of Science/Kavli Scholars program.
In addition, Dr. Datta is a cofounder of Optogenix (which manufactures biocompatible optical fibers for recording/manipulation of the brain), Syllable Life Sciences (which has developed an advanced behavioral phenotyping platform for drug development) and Abelian Therapeutics (which is developing novel treatments for neurodegenerative disorders).