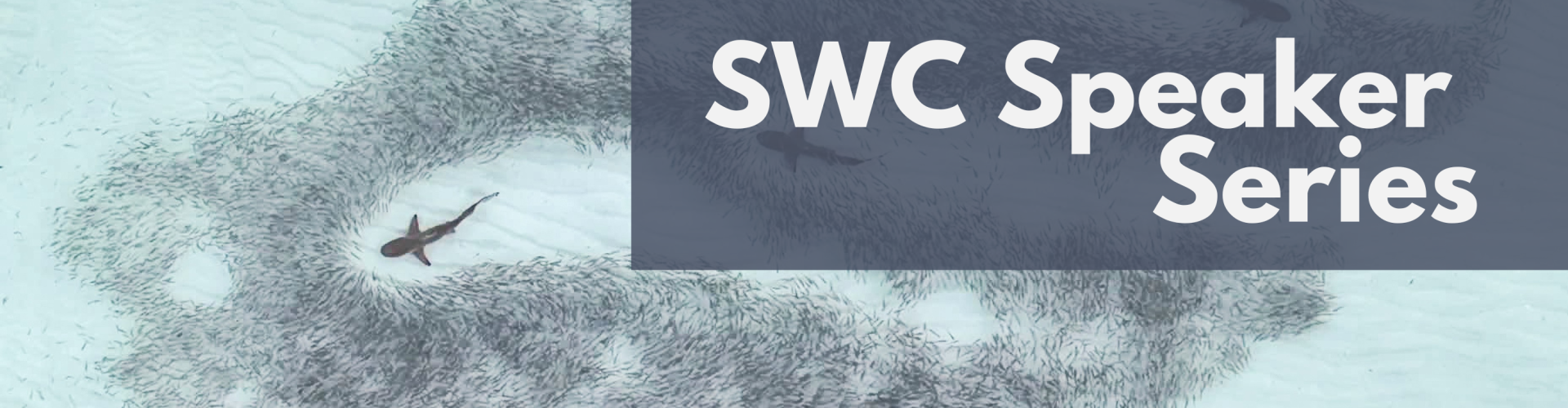
Exploring the geometry of decision-making
An interview with Professor Iain Couzin, Director, Max Planck Institute of Animal Behavior & Centre for the Advanced Study of Collective Behaviour, University of Konstanz, conducted by April Cashin-Garbutt
From synchronised starlings in the sky to the coordinated actions of ants, nature is truly mesmerising. This inherent beauty is what first sparked Professor Iain Couzin’s interest in collective behaviour and led him to study its fundamental principles. In a recent SWC seminar, Professor Couzin presented his latest findings on the geometric principles of decision-making. In this Q&A he shares how these insights could impact real world problems from robotics to crowd control.
What first sparked your interest in collective behaviour?
I've always been fascinated by animals. As a kid, I would collect bones, feathers, and insects, and I was particularly drawn to the behaviour of animals and the rich diversity we see. I was also really interested in ants—how these tiny creatures live within complex societies, coordinating together. It's inherently fascinating.
I'm also very interested in the visual arts, and my initial interest was more about the beauty of nature. The beauty of flocking and schooling is mesmerising—the balance between order and disorder is captivating. It's the diversity and beauty of biology that makes behaviour inherently fascinating and interesting.
Animals are constantly making decisions on the move, as they run, swim, or fly through the world. Why have many studies not focused on motion in decision-making?
There's been a preconception that motion is merely the output of a decision, but in many scenarios, feedback is very important. Animals constantly update their initial desires based on experiences through movement. And so, it is surprising that there were almost no studies reporting the movement of animals when deciding between spatially-discrete alternative options.
Recording and storing these movements was challenging, but with digital video and computation, we can now keep a record of rich data. For example, today I was watching some wonderful data on pairs of mice making decisions and I think their kinematics and movement is going to be really important for gaining insights into how the brain makes decisions.
Can you give an overview of your research on spatial decision-making and explain why you chose to study fruit flies, locusts and zebrafish?
We're very interested in trying to understand whether there are principles of spatiotemporal decision-making. We chose fruit flies and zebrafish because they are model systems—a model vertebrate and a model invertebrate—with accessible neural architecture.
Locusts were chosen because they are a major pest and we wanted to understand how they make spatial decisions. These plague insects can impact the livelihood of one in ten people on the planet, and so if we can understand how their brains make spatial decisions, there’s the potential to be able to apply this to help with control measures.
How have you been using virtual reality to study the decision-making of locusts?
Virtual reality (VR) is an important technology because it allows us to decouple things that are coupled in the real world. We can control and play with basic principles like how animals represent space and time.
VR also enables us to test theories explicitly, creating a feedback loop between theory and experiment, which is crucial for understanding the causal structure of decision-making.
What new and fundamental geometrical principles have you discovered and how do these impact decision-making?
Neuroscientists have found ring attractor dynamics in various systems, but these dynamics hadn't been studied in the context of a moving animal making decisions. As animals move, the geometry of objects with respect to the animal changes. This alters the neural representations of the objects, which changes the consensus dynamic about where the animal wants to move next, which changes the geometry and so forth.
This embodied decision-making introduces complex phenomenology, especially with multiple options. There has been a tendency in psychology and behavioural neuroscience to look at two options because it is relatively easy to do. But in the real world, animals have to deal with unpredictable and highly complex environments where there can be many options that they need to choose between.
For example, if a fruit fly is trying to find fruit, there may be an initial decision to move towards one tree or another, but when they get closer to the tree, suddenly there may be decisions at a more local scale. And so, it makes sense for a decision-making system to be inherently fractal and multi-scale.
We wanted to understand how the brain deals with this sort of complexity. We found that the brain warps space in its neural representation, which is critical for making effective decisions in complex scenarios.
How does the mechanism you found facilitate effective decision-making?
Even when an animal is making a choice, for example a fish following an individual, it still has a neural representation of the other individual. And so, if you get to an option and it is not as good as you expected, you can simply switch to the next best option. You don’t need a different model for each component.
It’s exciting that a very simple generative model can explain a wide range of experimental data from how animals search when they don’t have any information in their environments, to how they behave when they have complex environments with multiple options that they need to decide between.
Were you surprised by any of your findings?
I'm always surprised by our findings! For example, we recently published a paper that showed that the whole foundations of collective behaviour are on shaky ground because we had the wrong model at the level of the individual. It is surprising that a whole field can be misdirected in this way. It seems obvious in retrospect that collective motion can readily evolve from neural circuits involved in spatial navigation.
This realisation informs us in two ways. Firstly, we can make quantitative predictions for the first time, and secondly it gives us avenues to look at the evolution of sociality. It might be much easier to evolve to be social, or non-social, or to switch between the two, than we previously thought. This one model can fit a wide range of species.
We're just scratching the surface and are now working with mathematicians at Oxford and Cornell to understand how animals warp space, which is important for constraining the possible trajectories animals may experience.
Is it likely that humans use the same geometric principles?
I’m always cautious about humans, because people are quite exceptional in many cases, but we first discovered there's a deep mathematical connection between flocking models and ring attractor models. By studying the ring attractor, we realised that the ingredients of the flocking model were wrong.
In some sense, we're at the very beginning of understanding how this coupling works, but working with mathematicians, we've been able to show that models used in different fields, including humans, have the same fundamental dynamical properties. This helps us design experiments and to understand the principles to look for.
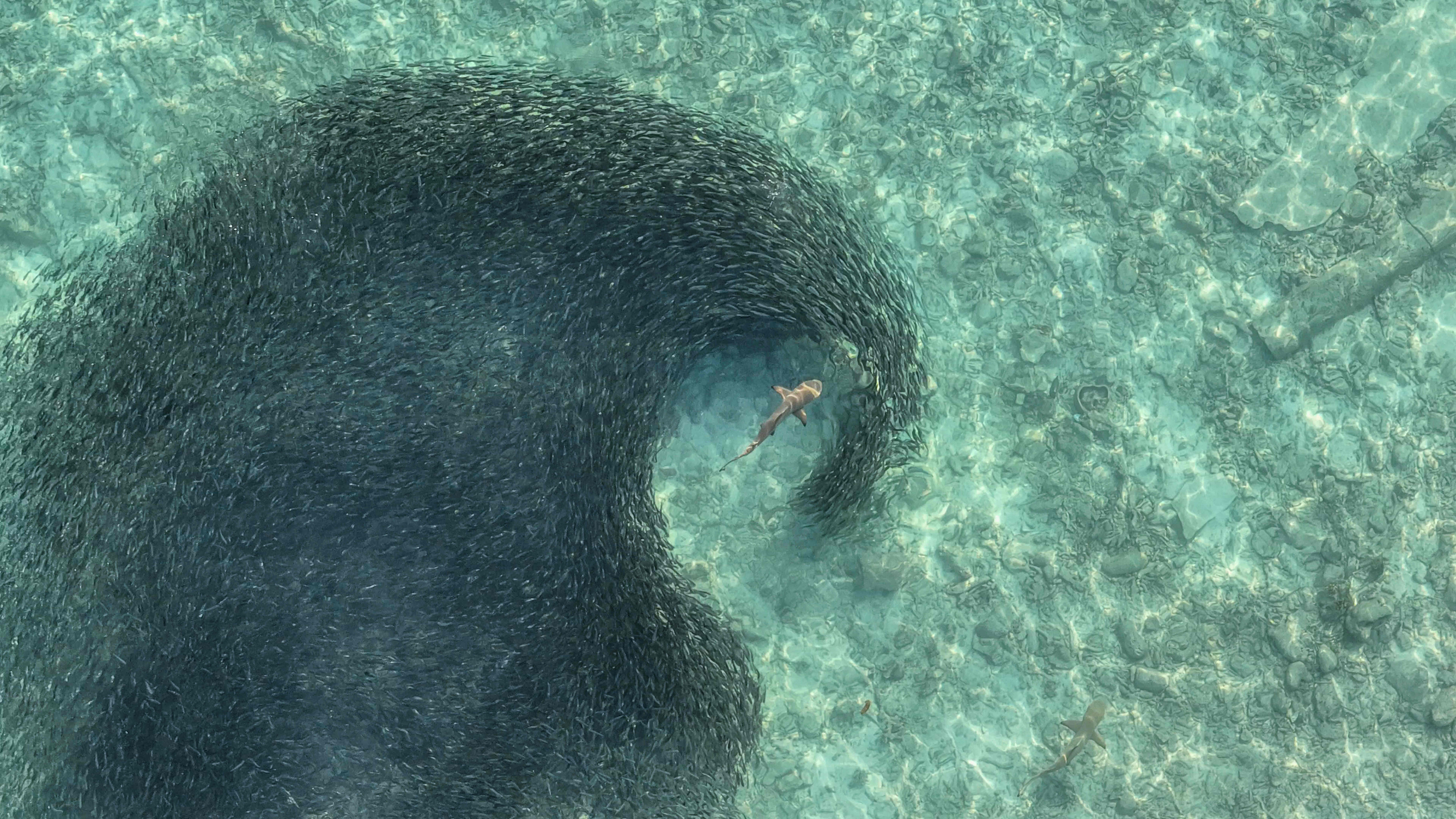
What are the main implications of your findings for real world problems?
There are multiple potential implications. Preliminary data suggests that some ingredients of this decision-making process are involved in humans making spatial decisions as they’re walking through space. This could enhance models of crowd control or pedestrian dynamics.
There are also implications for robotics. If we want to make robots that make smart decisions while moving through space and that can coordinate their behaviour with each other, then we can learn from animals. The evolved strategies used by animals could be implemented as control laws directly in robots. This is a powerful and meaningful approach that works in the real world. We are getting a lot of interest from the robotics community.
A third application is to apply our findings to animals that impact humans in a variety of ways. Understanding how animals coordinate behaviour can help predict swarms and control efforts, impacting food security and sustainability.
What is the next piece of the puzzle you hope to solve?
We're looking for fundamental mathematical predictions about spatiotemporal decision-making that transcend study systems. This could give us deeper insights into how decision-making functions.
I think there is a great benefit in being adaptive, utilising technologies and being malleable. We shouldn’t stick to old models, even when we have developed them, but embrace new ways of seeing the world.
I find this multidisciplinary perspective exhilarating. We don’t know what is coming next, we don’t know what accidental discoveries we might have next week. That’s the great thing about running a lab – there are always many chance observations that can lead to a redirection in our research.
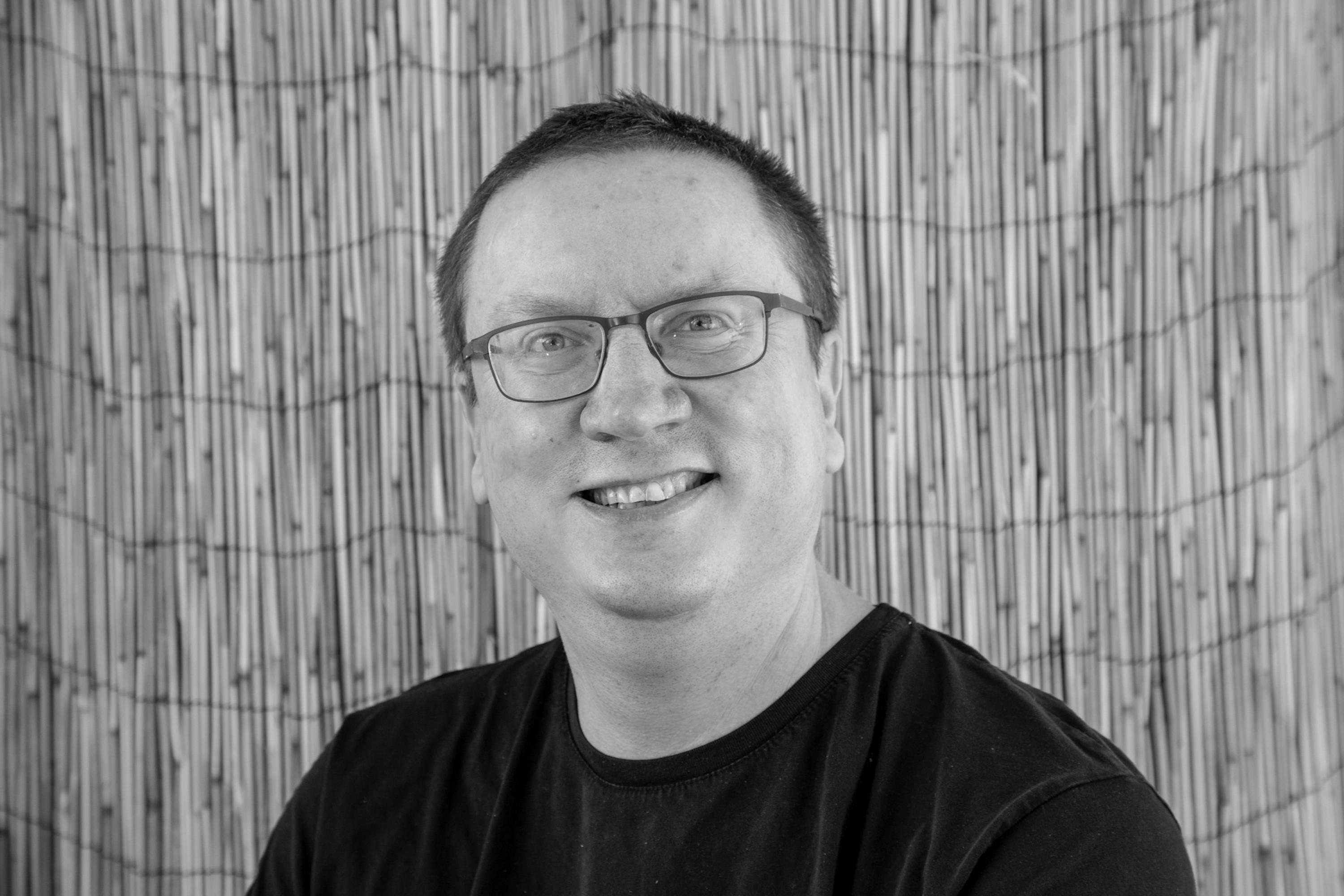
About Professor Iain Couzin
Iain Couzin is Director of the Max Planck Institute of Animal Behaviour and a Professor and Director (Speaker) of the German Research Foundation (DFG) Excellence Cluster “Centre for the Advanced Study of Collective Behaviour” at the University of Konstanz, Germany. Previously he was a full Professor in the Department of Ecology and Evolutionary Biology at Princeton University. His work aims to reveal the fundamental principles that underlie evolved collective behaviour, and consequently his research includes the study of a wide range of biological systems, from neural collectives to insect swarms, fish schools and primate groups. In recognition of his research, he has been the recipient of the Searle Scholar Award (2008); one of the top five most cited papers of the decade in animal behaviour research (1999–2010); the National Geographic Emerging Explorer Award (2012); the Scientific Medal of the Zoological Society of London (2013); named a Web of Science Global Highly Cited Researcher (2018–2022 and 2024); the Lagrange Prize (for fundamental contributions to complexity science, 2019); the Falling Walls Life Sciences Award and the Gottfried Wilhelm Leibniz Prize (Germany’s highest research honour) (2022); the Rothschild Distinguished Fellowship at the University of Cambridge (2023); the Fyssen International Prize (2024); and the President’s Medal of the Royal Society of Entomology (2025).
Banner image credit: August Paula and Angela Albi