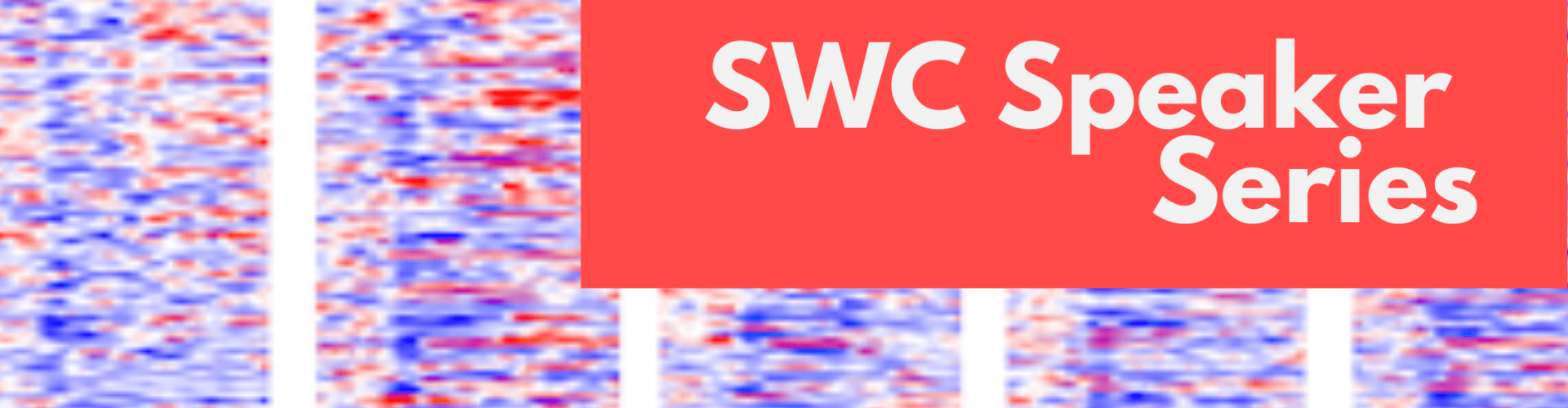
Learning and forgetting in primary olfactory cortex
An interview with Dr Carl Schoonover and Dr Andrew Fink, Postdoctoral Fellows in the Axel Lab at Columbia University, conducted by April Cashin-Garbutt
If you place a mouse into a box, it doesn’t just sit there – it sniffs around and checks out its new environment. No rewards are needed to see this behaviour; the mouse does this simply because there is something in the world instead of nothing.
As the mouse investigates, it learns about the structure of the world so it can build an internal model to successfully navigate. But how does the brain learn about such odours and how often are these memories overwritten? I spoke with Dr Carl Schoonover and Dr Andrew Fink, who recently gave the final lecture in our 2020 Emerging Neuroscientists Seminar Series, to find out more about learning and forgetting in primary olfactory cortex.
How does your work differ from traditional olfactory learning studies?
Dr Andrew Fink: While a lot of work has been done on learning, there is still so much to do. Our specific motivation was to try to find a way to study learning outside the standard Pavlovian and operant conditioning approaches because we suspect that the reinforcers required in those paradigms have profound effects on the learning process and the underlying neuronal mechanisms.
Dr Carl Schoonover: Pavlov paired the sound of a bell with a meal and asked how the representation of the bell sound gets paired with, or becomes predictive of, a good outcome. The question that Andrew and I are going after is: what is the dog learning about the bell?
We argue that most biological learning is not based on explicit pairing with a reinforcer, and we would like to isolate that kind of learning because it may operate quite differently from more commonly studied forms. However, it is difficult to access this behaviourally because it is really useful to have something like a drip of saliva coming out of a dog’s mouth as a way to indicate that the animal is able to predict the outcome of the bell sound. If you don’t have something like a conditioned response, it becomes very difficult to infer that the animal has learned something and also difficult to measure the rate at which it’s learning.
As a result, the forms of learning that have been most accessible to experimental psychology and behavioural neuroscience are very specialised. The vast amount of learning that isn’t based on explicit reinforcement has been relatively understudied because it is difficult to access behaviourally.
Why is it interesting to study the exploratory behaviour of animals in new environments?
Dr Carl Schoonover: It is maladaptive for an animal to explore all of the time. It simply is not possible to form a complete model of the world and there are competing drivers that the animal needs to balance, such as avoiding predators. This raises a basic biological question: when and why do animals stop exploring a new environment? They stop long before they have formed a complete model of what they are exploring, but what is it about the model that makes it good enough?

Activity from an individual neuron recorded in the primary olfactory cortex of mouse, as it responds to eight different odours (columns) on five separate days (colours) across approximately one month. Each small tick indicates an individual spike of voltage, each thin row an individual trial. This neuron was found to encode the eight odours in the same way across a period of 32 days.
For how long has the piriform, or primary olfactory, cortex been hypothesised to facilitate the identification of odorants?
Dr Andrew Fink: In 1941, E. D. Adrian was working at the Physiological Laboratory, Cambridge, and he observed odour responses in piriform using his method of recording. Then in 1969, Lewis Haberly recorded in piriform and saw odour-evoked responses, so people knew that the system responds to odorants.
But interestingly, it wasn’t until this century that it became possible to observe enough activity to start to put together the hypothesis that piriform is encoding odour identity. The reason is that piriform is not like the primary visual cortex (V1) where you can do a Hubel and Wiesel type of experiment and present a continuum of stimuli (such as lines at varying orientations) and define the response properties of the neuron to that family of stimuli. In piriform, if you were to try to do this, you would have to present one odorant followed by another and another and there would be no way to tell what’s going to happen based on the previous ones (unless they are very similar). People needed to observe large populations of cells to see that the cortex is responding to odour in a distributed way.
Why would we expect piriform to produce a readout that varies little over an animal’s lifetime?
Dr Carl Schoonover: If the role of the region is to identify odours, for example to identify that banana came in at the nose, the easiest way to achieve this is to have a reasonably stable response to those stimuli (over the animal’s lifetime) that the rest of the brain can use. This is more or less what has been observed in primary visual cortex, auditory cortex, and somatosensory cortex. People have found that in these neocortical regions either there is no drift at all, or if there is any, it is extremely slow as compared to the lifetime of the animal.
The intuition is that this stability is an advantage if the purpose of the region is to broadcast to the rest of the brain what is coming in at the periphery. If it isn’t stable, then there needs to be some kind of compensatory mechanism to deal with the fact that it’s drifting over time. This adds a layer of complexity to the system, that we argue is unnecessary in the case of the olfactory system because the olfactory bulb may in principle be able to do a perfectly good job on its own.
Dr Andrew Fink: The first order model of piriform when we started this project was that you have the bulb, which has the glomeruli and odours stimulate the patterns of activity involved. But there’s some overlap between the patterns, they’re not perfectly distinct, and so the role of piriform is to expand the bulb’s relatively low-dimensional representation to a high-dimensional representation such that every odorant may then evoke a pattern of activity that’s very readily discriminate from every other odorant.
And indeed this enormous cortex facilitates very easy discrimination between stimuli. However, a second ingredient, if piriform activity, on its own, establishes the identity of odorants is that its responses to odours be reasonably stable over time.
What strategy did you develop to measure odour tuning over time?
Dr Andrew Fink: The best way to measure neuronal activity over time is to employ a two-photon microscope to look at a sheet of cells and track responses over time. That’s not an option for us because to get that sheet of cells you have to remove the jaw of the mouse.
Instead we developed an electrophysiological method. Our approach to track cells over time using electrical recordings relied on three basic principles. The first was to have as many electrodes as possible in as small a place as possible to have as many “eyes” on each spike as we could. So we used the silicon probe with the densest site spacing that was available when we started these experiments.
The second principle is to use silicon probes that have a very small footprint. The probe we use has a single shank and we insert it into the brain in such a way as to minimise damage to the tissue. The probe is precisely aligned to its axis of travel so it is perfectly straight and the footprint it makes in the brain is 15 microns thick.
The third principle is that we don’t use a microdrive, instead we anchor the probe in place so that we ensure it moves as little as possible with respect to the tissue. After implantation we wait for several weeks to allow the brain to settle around the probe and for things to stabilise. We only start taking data about odour responses once we’ve established that we can follow a set of single units every day.
There are a lot failure points, and it took several years to solve this.
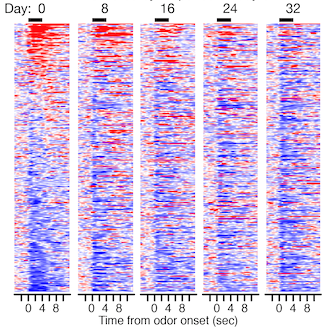
Activity from 300 individual neurons recorded in the primary olfactory cortex of mouse as they respond to odour, sorted on day 0 according to increases (red) and decreases (blue) in spiking. Each row corresponds to one neuron; the odour is presented for four seconds beginning at X=0. Maintaining the same sorting on subsequent days shows that the response of most neurons gradually changes such that by day 32 it is nearly completely different.
What key observations did you make and what surprised you the most?
Dr Carl Schoonover: Our first observation was drift in the piriform. We were not entirely surprised by this—in fact we invested all this time and energy solving the acquisition problems because we predicted there should be drift based on the anatomy of piriform, the logic of the circuit and one assumption: that synapses in the cortex are plastic. However the anatomy did not predict the rate at which it would drift. It turns out that it drifts quite rapidly, which was surprising to us. And we’re lucky that our limited window of observation (approximately one month) was sufficient to catch it.
Our two other main results (regular experience stabilises responses, but only so long as regular experience is maintained) were surprising, in that we had no way to predict them a priori. In combination with the classical conditioning result, these brought us to our current interpretation of the data: that this system is remarkably plastic, that it can learn and encode memory traces of some kind, but that it is not able to hang onto them over the long term.
Dr Andrew Fink: For me, there were two phases of surprise. The first was seeing how plastic piriform could be and how malleable odour responses could be both as a function of time and experience. For a while, this led us to this idea that piriform is just a learning system.
Then the second delightful surprise for me was to realise that learning is a really intimate component of perception, and that the more we thought about this system, the more the idea of it only as a purely sensory or a purely learning system went away. I don’t think the ideas of piriform being a sensory system or a learning system are opposed at all.
What predictions does this view produce and how will you test them?
Dr Carl Schoonover: There are some very specific predictions that can be made. For example, if our interpretation is correct and we were to place a cohort of animals in an environment that was very odour-rich, we predict that the rate of drift would go up as we’d expect more overwriting to occur. If we could make this observation, then this would confirm our interpretation that the system is continuously overwriting itself as a function of new experience.
Dr Andrew Fink: Basically, the more the animal has to be learning, the more overwriting there is and the more drift there is.
Dr Carl Schoonover: This would be a nice general confirmation of an idea that’s been in the field, especially in the theoretical literature, that as a system learns it overwrites itself, unless there is a mechanism to counteract that. And so if we could make an empirical observation of exactly that, and learn about the underlying neuronal mechanisms, then I think it would have value beyond just what the piriform is doing in the context of olfaction.
We also have a gut feeling about what we’re dealing with in piriform: what we think piriform is offering us is a relatively simple model of the CA3 region of hippocampus. If correct, this presents several practical benefits. In particular, the inputs to piriform are fairly straightforward to control, either by using odours or more sophisticated methods like optogenetic activation of cells in the olfactory bulb.
We are working to establish whether this gut feeling is reasonable. If it is, then the field may be in a position to study aspects of the function of a well-known associative circuit like CA3, but under conditions over which we have fairly good control. There is a lot more to test, but as we lay down the groundwork for this research program we hope to make more general statements about the function of associative cortices.
About Dr Carl Schoonover and Dr Andrew Fink
In graduate school, Carl Schoonover (BA Philosophy, Harvard College) studied the thalamocortical projection to primary somatosensory cortex under the supervision of Randy Bruno, and Andrew Fink (BA Physics, Carleton College) studied spinal presynaptic inhibition under the supervision of Tom Jessell.
Andrew and Carl formed a close collaboration upon joining Richard Axel's lab at Columbia University for their postdoctoral fellowships, during which they have laid the foundations of a joint research program to investigate the neuronal mechanisms underlying non-reinforced learning. They have developed a behavioural assay for the mouse that yields a readout of a subject's ongoing learning of the statistics of olfactory scenes. In parallel they have developed electrophysiological methods to observe the spiking activity of large populations of single units over extended periods of time. This has revealed the primary olfactory cortex to be exquisitely sensitive to the statistics of recent odour stimulus history, and to be capable of predicting future olfactory events based on past regularities.