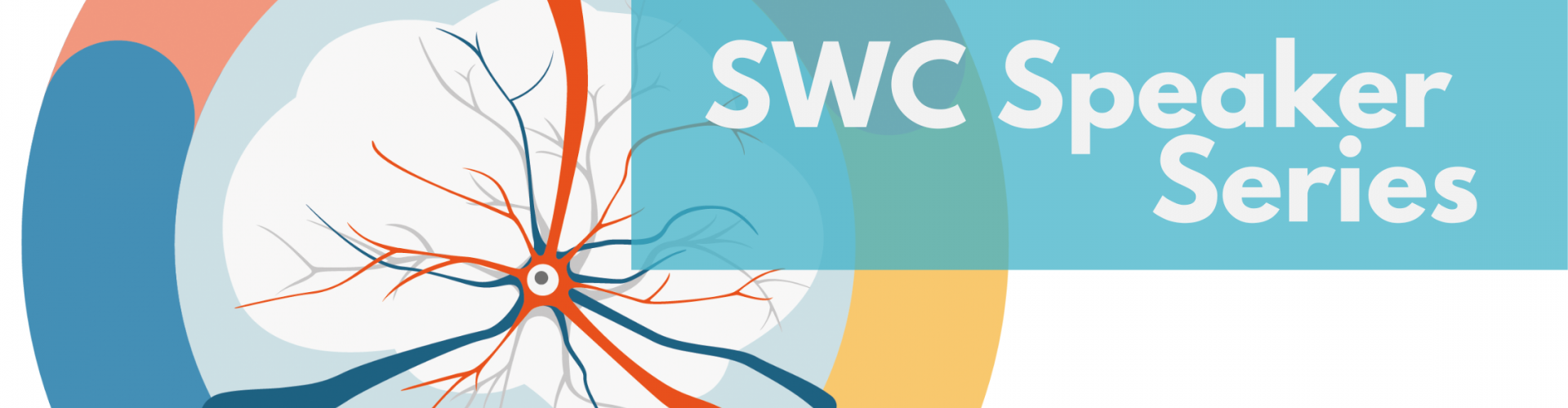
Simple pleasures: regulating feeding and social interactions
An interview with Professor Tatiana Korotkova, Institute of Physiology, University of Cologne conducted by April Cashin-Garbutt
How does the brain coordinate multiple, and sometimes competing, basic needs? In a SWC Seminar, Professor Tatiana Korotkova shared her research on regulating behaviours like feeding and social interactions. In this Q&A, she touches on why her lab focuses on the lateral hypothalamus, the techniques they are using to investigate and some surprising findings to date.
How much is known about the way animals coordinate and prioritise multiple, and sometimes competing, needs to ensure survival and reproduction?
We don’t know much about this as usually we study one behaviour at a time. Traditionally, we hypothesised that one particular cell type would be responsible for one particular behaviour. However, we now think that the same cells may control different functions and behaviours and coordinate them. We are now using a behavioural paradigm where we are analysing the response of the same cells to different innate rewards.
Until recently, it was not possible to answer these questions in systems neuroscience because we didn’t have the right methods. The knowledge from classical studies was gained using electrical stimulation and lesion experiments, but these weren’t cell-type specific. They provided information on the main functions of particular brain regions but they didn’t provide us with details. Advances in technology have allowed us to record neural activity in freely behaving animals and also to manipulate neural activity using techniques such as optogenetics.
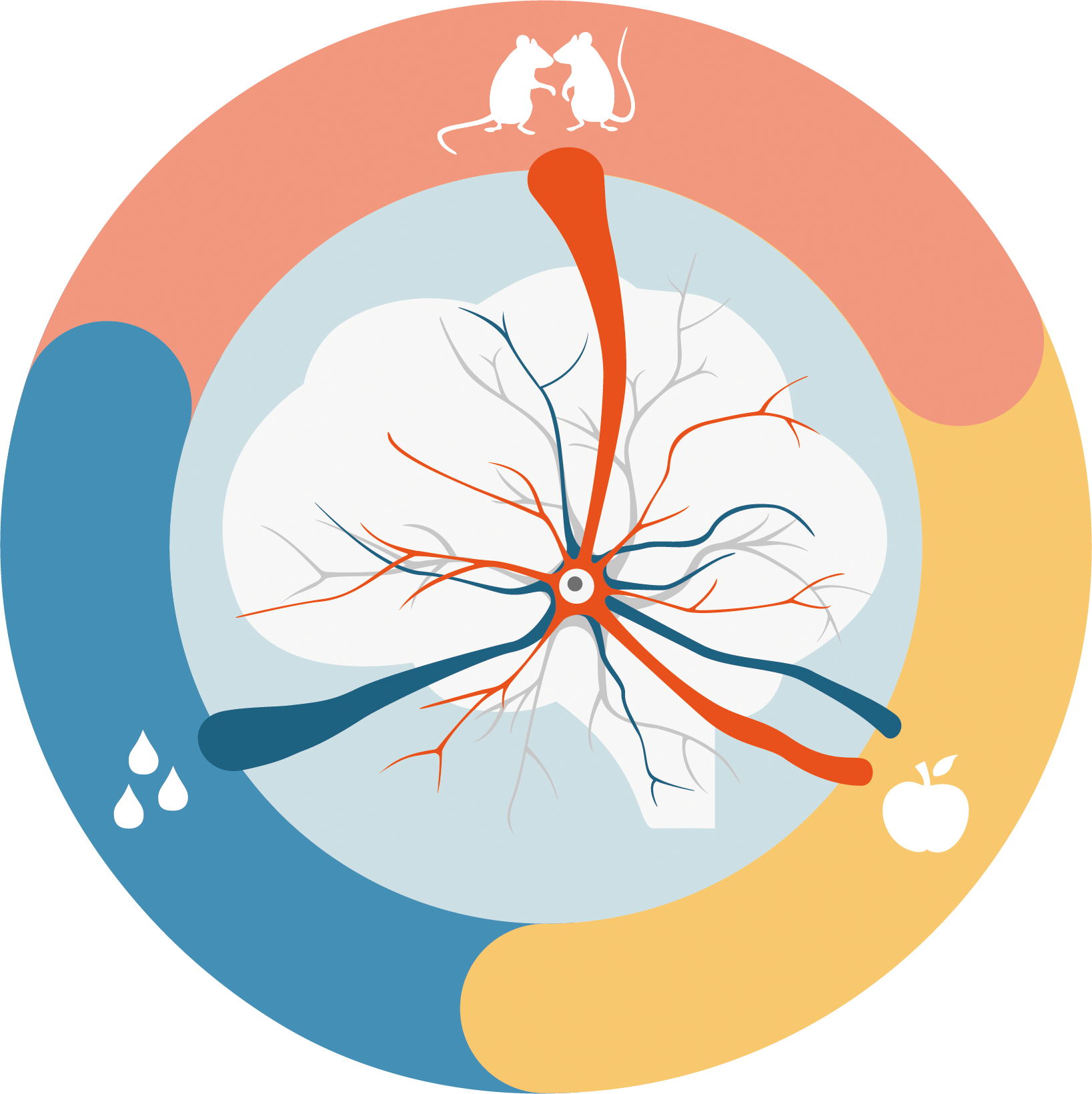
Why does your lab focus on the lateral hypothalamus in the brain?
During my PhD, I studied the actions of hypothalamic neuropeptides on the reward system - dopaminergic neurons in the ventral tegmental area (VTA). That’s when I became interested in the lateral hypothalamus (LH) but at that time the necessary methods to study neuronal activity of identified LH neurons in freely behaving mice weren’t available. I performed experiments on brain slices, where I applied different neuropeptides that are expressed in LH on the VTA slices.
I switched to in vivo experiments during my postdoc in the Monyer lab and at that time the vast majority of in vivo studies were performed in the hippocampus. Then as a junior Group Leader I thought more about what research questions I wanted to tackle and I wanted to combine my postdoctoral expertise with my initial interest in the hypothalamus and hypothalamic circuits. And so I decided to study the top-down inputs from hippocampus and prefrontal cortex to the lateral hypothalamus.
The lateral hypothalamus is fascinating because you have so many different cell types and many of the neighbouring cells have opposing functions. We now have many tools that we can use to study this because there are multiple CRE mouse lines available for hypothalamus. For example, to be able to report or manipulate activity we need to be able to express opsins in a particular cell type.
Can you describe the techniques you are using to investigate the functions of neuronal populations in LH that are involved in feeding and social interaction?
We perform neural recordings in freely behaving mice. We use either electrophysiology or calcium imaging. To do so we implant electrodes, called silicon probes, in the case of electrophysiology, or we implant GRIN lenses for calcium imaging, that mice can live with for several weeks or months. This is similar to people with Parkinson’s disease who do not respond to pharmacological treatments anymore and also receive chronic electrodes that stimulate parts of their brain so that they don’t experience symptoms such as tremor.
We record the neural activity of cells in a given brain region as mice spontaneously behave. For example, we have a behavioural enclosure where mice have access to food, water, other mice and a novel object. We record the behaviour of the mice and the neural activity at the same time. These two flows are synchronised so you can analyses the changes of activity prior to behaviours, during behaviours and afterwards. This is how we define cells as food-related or social-related cells for example. We can look at things like how diet changes the priorities and coding in the brain.
For manipulation of neural activity, we use optogenetics. To do so we express opsins in cell-types of interest. Opsins are light-sensitive channels. This means we can activate or inhibit those cells in behaving mice, which allows us to gain causal evidence.
We can also combine these methods, so we can record cells during spontaneous behaviour and then we can manipulate activity and record during manipulation. Then we see which manipulations we elicit, how much of an increase in firing rate there is and what changes in behaviour we observe.
What have been your key findings so far?
In the context of hypothalamus, we discovered the circuit that promotes food approach and we disentangled the different components of circuits that promote food approach and regulate food intake. We also found which changes occur in the neural dynamics in the lateral hypothalamus when you decide to approach food or not approach food and when you decide whether or not to eat the food (Carus-Cadavieco et al., Nature 2017).
The original concept was that food approach and food intake are more or less the consequent stages of the same behaviour, as you have to approach food to eat it, but sometimes those behaviours are also disentangled. For example, sometimes you might walk to the fridge and open the door but then close it again. Sometimes animals hunt but then don’t eat the prey. Sometimes animals, like squirrels, hoard food and don’t eat it for a while.
We are now looking at subtypes of GABAergic cells in different aspects of feeding and social behaviours. Surprisingly we found that feeding and social behaviours are modulated by the same cell types in the lateral hypothalamus.
Can you please explain why you focused on leptin receptor-expressing neurons and neurotensin-expressing neurons?
In the food approach study, we worked on functions of GABAergic cells in the lateral hypothalamus and the top-down inputs onto those cells. But then when we analysed activity of those cells in response to feeding, we found that we still had subgroups within these GABAergic cells. Some cells responded to feeding with an increase in firing rate and others responded with a decrease in firing rate. Some cells didn’t respond at the beginning of feeding but then they responded as the animal became more satiated.
We decided to explore these different subgroups further and we concentrated on leptin receptor-expressing cells because leptin is a hormone released by fat cells and so is a satiety signal. Neurotensin is a neuropeptide that is also involved in food intake but the function was not 100% clear from previous studies.
Were you surprised to find leptin receptor-expressing neurons prioritise social interaction despite acute hunger or thirst?
Yes, this was our most surprising finding of the entire project! Anne Petzold, a postdoc in my lab, initially used a social stimulus as a control innate reward, and the innate reward, which we actually studied, was food. We did not expect to see so many cells respond to the social stimulus.
Did you find differences in male and female mice?
Yes, we found that those cells increase their activity when mice interact with a mouse of opposite sex – in males they are excited when they interact with females but not with males, in females – during interaction with males but not with other females. Further, we saw was that the activation of those cells increased social interactions with females compared to males. This was also very surprising.
Were you surprised to find neurotensin-expressing neurons specifically promote drinking despite hunger and reduce social interactions?
It was already known from previous studies that these neurons can promote drinking, but this was surprising in our experiment as our hypothesis was that they would follow the need state. When they are hungry, we would expect these neurons to promote eating. However, water is a very crucial need that is vital for survival. Even when you are not hungry, you should keep your water source in mind because you don’t have limitless access to water. And so the mouse should always make sure the water source is not too far away.
This is the great thing about neuroscience. We don’t just do experiments to confirm our hypotheses; we discover new things all the time.
What are the main implications of your research?
The main implication is the understanding of neural mechanisms that drive our basic motivations. If you think about neuro-psychiatric diseases, they are mainly discoordination of cognition and motivation and emotion.
For example, the alteration of feeding behaviours results in a whole range of eating disorders from anorexia to obesity. These conditions are widely spread and difficult to treat. Anorexia has the highest mortality rate of all psychiatric diseases with a 20% mortality rate. That’s why I think understanding how feeding is regulated can gain insight into how different therapies can be developed.
For anorexia, the only therapy at the moment is cognitive behavioural therapy (CBT) and there are no pharmacological treatments. The development of such treatments would be very beneficial. The same is true for obesity, where pharmacological treatments are limited.
There is also the problem of high comorbidity of psychiatric diseases. For example, anorexia has high comorbidity with anxiety disorders and social phobias, which is interesting as we see that e.g. lateral hypothalamus regulates both feeding and social behaviours.
There is also a connection between feeding and locomotion. During the development of anorexia, patients eat less and less and they move more and more as they undertake voluntary exercise and lose weight even faster. In obesity, this is the opposite. Patients move less and less, they lose motivation to move and physically it gets more difficult.
I hope that understanding how these innate behaviours are interconnected will help to tune the motivation in a more healthy way. It will also be important in the context of healthy ageing. Obesity often occurs fairly early during ageing and these regulations promote vicious circles that have add-on effects. And so understanding how these behaviours are coordinated and prioritised will help us to help individuals.
What’s the next piece of the puzzle your research is going to focus on?
We are going to look at the encoding of different innate behaviours by the same neurons or neighbouring cell types. We will try to understand the underlying circuits through studying the inputs and outputs of the cells and how they execute the different aspects of different behaviours.
An interesting thing about the lateral hypothalamus is that, compared to other hypothalamic nuclei, it has huge numbers of inputs and outputs with distant brain areas. For example, it has direct inputs from the prefrontal cortex, which can execute cognitive control over hypothalamus. This can be good by helping us not to overeat, but it can also be involved in conditions like anorexia due to the increased cognitive control.
Where can readers find out more?
In our paper on LepR and Nts cells:
Anne Petzold, Hanna Elin van den Munkhof, Rebecca Figge-Schlensok, Tatiana Korotkova. Complementary lateral hypothalamic populations resist hunger pressure to balance nutritional and social needs (2023) Cell Metabolism 35, 1-16. https://doi.org/10.1016/j.cmet.2023.02.008
This work was also recently featured in Nature News: Sex, food or water? How mice decide
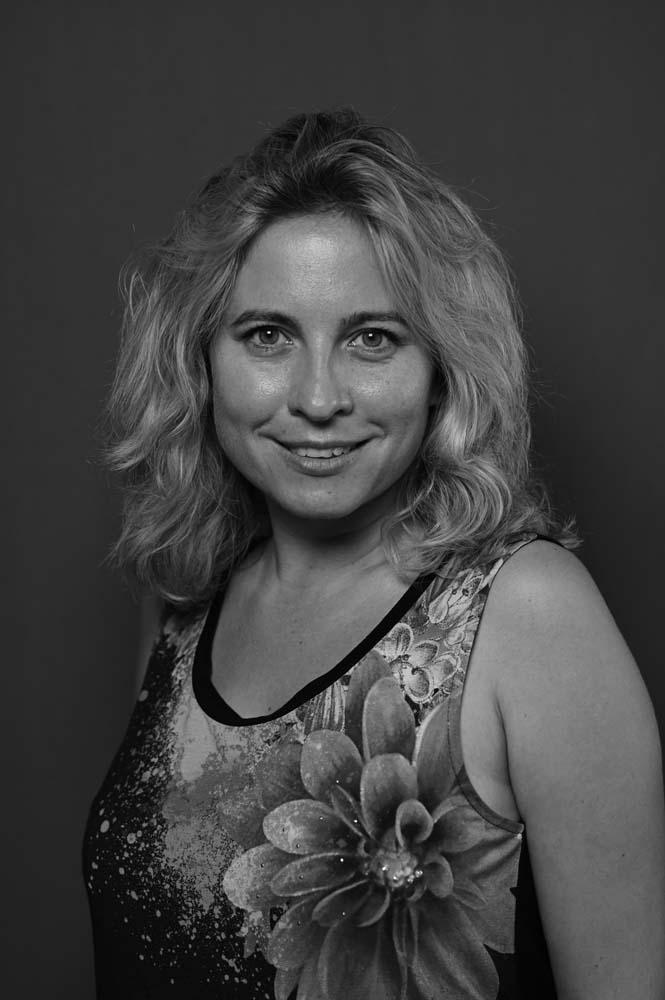
About Professor Tatiana Korotkova
Tatiana Korotkova studied biology with a focus on human and animal physiology in Lomonosov Moscow State University. During her Ph.D. under the supervision of Dr. R.E. Brown and Prof. H.L. Haas in Düsseldorf she investigated actions of various hypothalamic neuropeptides on aminergic nuclei, particularly on dopaminergic system. As a postdoc in the lab of Prof H. Monyer in Heidelberg, and later in a collaboration with Prof. T. J. Jentsch in Berlin she studied mechanisms of hippocampal network oscillations in behaving transgenic mice. In 2012-2017 T. Korotkova was a junior group leader at the Leibniz Institute of Molecular Pharmacology (FMP)/Neurocure Cluster of Excellence in Berlin, in 2017-2019 - a research group leader at MPI for Metabolism Research. Since 2019 T.Korotkova is a Full Professor and Managing Director at the Institute of Physiology, University of Cologne. She was a holder of the DFG and Schering foundation research stipends, and was awarded the Human Frontier Science Program (HFSP) Grant as well as the Junior Brain Prize by Lundbeck Foundation. The group of Tatiana Korotkova investigates neuronal mechanisms of innate behaviours, including feeding, social interactions and voluntary locomotion, in health and disease. Her research is supported by the European Research Council and by the German Research Organization (DFG). She supervised 10 Ph.D. and 12 M.Sc. students, and co-authored 30 peer-reviewed original publications, 3 reviews as well as one book chapter.