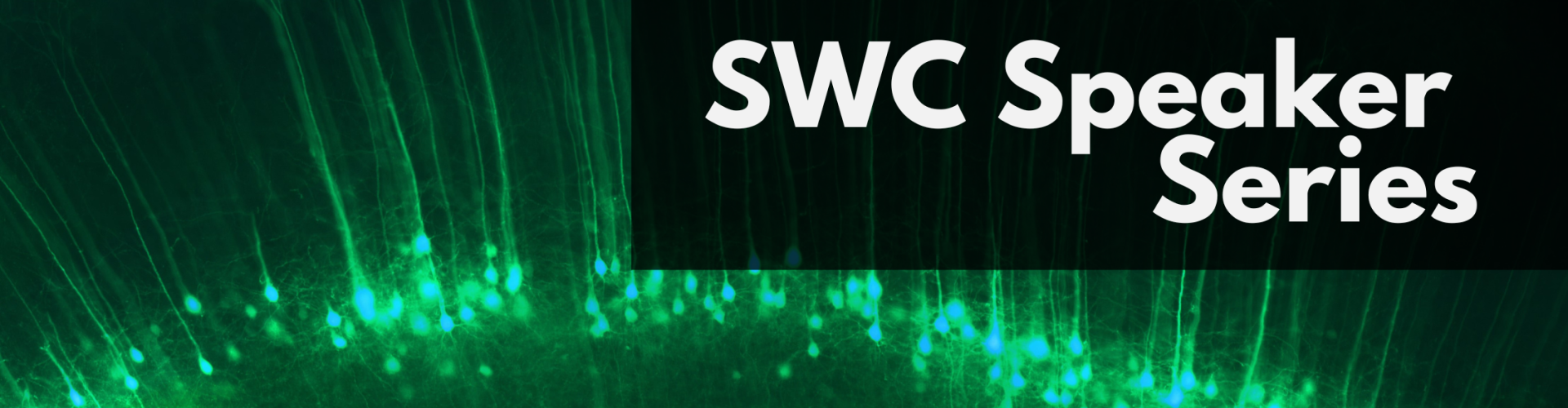
Synapse pruning in development and Alzheimer’s
An interview with Professor Carla Shatz, Stanford University & Bio-X, conducted by April Cashin-Garbutt
How can understanding synapse pruning in development help tackle neurodegenerative disease? In a recent SWC Seminar, Professor Carla Shatz demonstrated how looking at fundamental questions in neuroscience can lead to important discoveries about diseases such as Alzheimer’s.
In this Q&A, Professor Shatz shares why the connection between Paired immunoglobulin-like receptor B (PirB) in normal development and disease took quite a while to emerge, and the implications for synapse pruning disorders.
You have devoted your career to understanding the dynamic interplay between genes and the environment that shapes brain circuits. What motivated you to pursue this path?
My original interest was in art, and I couldn't decide whether I wanted to pursue a career in the arts, delve into understanding visual perception, or go into science. It's important to note that when I began my journey, neuroscience wasn't yet established as a field! There were disciplines like psychology and physiology, but I ended up majoring in chemistry.
My fascination with art and visual perception grew during my undergraduate years at Harvard, particularly through the courses I took in painting and design. On the other hand, my scientific curiosity also thrived. Towards the end of my undergraduate studies, I had to choose a topic for my senior thesis. When my senior advisor, who was a chemistry professor, asked me what I wanted to do, I expressed my lack of enthusiasm for chemistry. He suggested I explore the labs of two young professors at Harvard Medical School who were just starting out. Their names were Hubel and Wiesel, and it turned out to be a perfect fit.
Hubel and Wiesel were conducting groundbreaking experiments on the central visual pathways, though they hadn't yet received the Nobel Prize for their work. I was fortunate enough to be the first undergraduate student to join their team. They were intrigued by the idea of having an undergrad in their lab, and it was an exciting opportunity for me as well. This experience marked the beginning of my journey into understanding how we perceive the world visually.
From that point on, the trajectory of my career was set and the rest, as they say, is history. It was a thrilling and formative experience for me.
How much is known about the way connections are wired up during brain development? How does this process differ prenatally and postnatally?
There's a wealth of knowledge we've gathered from animal models, particularly in understanding the earliest stages of brain wiring. Countless exquisite experiments, both genetic and observational, in organisms like drosophila, mice, and even cold-blooded vertebrates have shed light on this.
Essentially, there are two fundamental aspects of brain wiring that have emerged from this research. First, there's an initial phase of wiring that occurs independently of experience or neural activity. This phase is primarily governed by guidance molecules, which lay down the basic framework of brain circuits. Then, there's a subsequent phase that occurs later in development, which does rely on experience to refine and sculpt circuits based on our interaction with the world.
What's fascinating is that this later phase actually begins before birth, preceding any external experiences. This was a discovery made in my own lab, showing that early spontaneous neural activity is crucial for proper wiring, even before sensory inputs like vision are possible. It's this early activity that sets the stage for the intricate patterns of connectivity seen in the mature brain.
We've made significant progress in understanding the basics of how this wiring occurs, but there are still major mysteries. For instance, we're still grappling with how the growing axons from the neurons from the retina recognize and grow into their targets in the brain. The signals that guide this process remain largely unknown but are undoubtedly crucial not only for fundamental wiring questions but also for potential applications in nerve regeneration.
Furthermore, the extended periods of development after birth, where experience plays a critical role, are still not fully understood. While we grasp the general principles of synaptic remodelling and pruning, the regulation of these early developmental periods remains elusive. Different brain regions have their own critical periods, and the factors controlling the opening and closing of these periods are complex and multifaceted.
In terms of development-related questions, there's still a plethora of unknowns awaiting answers. These inquiries aren't just academic; they're crucial for understanding and potentially treating various diseases that afflict the brain, as well as spinal cord injury. So, while we've made significant strides, there's still much to uncover.
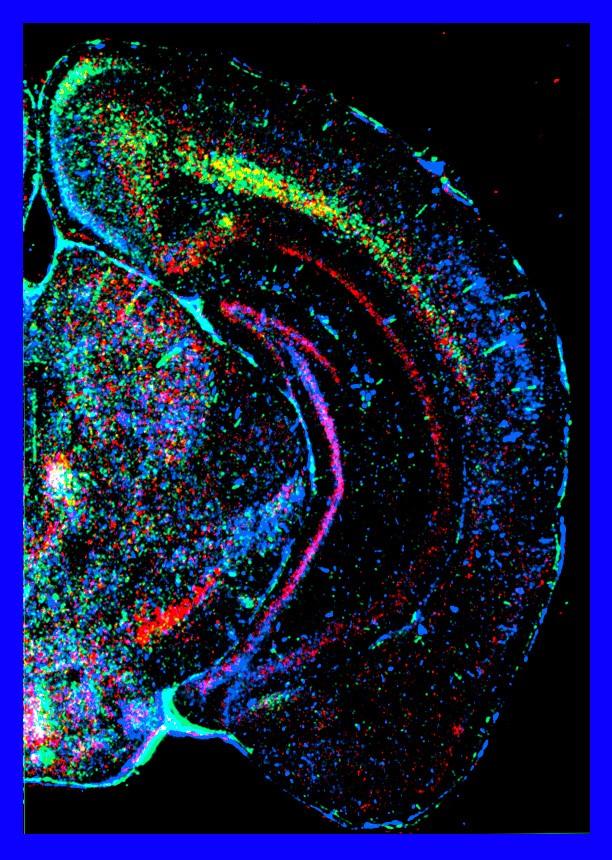
This image relates to work by Huh et al, Science 2000.
How does your research on genes such as Paired immunoglobulin-like receptor B (PirB) also link to Alzheimer’s disease?
The connection between PirB (called LilrB, leukocyte immunoglobulin-like receptor B, in humans) in normal development and disease took quite a while to emerge in my lab. This is because we were, and still are, very engaged in studying the normal function of this receptor and the interplay with various ligands such as Major Histocompatibility Class I and beta-amyloid.
The complexity of these ligands, coupled with their polymorphic nature, prompted us to explore PirB as a receptor. We found remarkable developmental phenotypes in the absence of PirB. When we knocked out the gene PirB, the mice seemed fine! This was puzzling and so we looked at whether or not this developmental critical period for vision was normal.
Hubel and Wiesel’s major discovery, that led them to their Nobel Prize in Physiology or Medicine in 1981, showed that there was an early critical period of development when using both eyes together is crucial for the formation of normal binocular function in visual cortex. When one eye experiences amblyopia, characterized by reduced visual acuity, it fails to develop robust connections to the cortex, while the other eye's input essentially dominates. This phenomenon results in a scarcity of binocular cells, posing a significant challenge to human visual development, especially our ability to perceive depth. Amblyopia affects an estimated 2 to 4% of people in the United States, underscoring the importance of early intervention.
Hubel and Wiesel’s research revealed a critical developmental period when during the first five or six years of human life, both eyes must be used together to establish highly functional binocular vision. This understanding prompted the creation of animal models to study visual development further. These animal models then led to the discovery that during this critical period in the cortex, extensive synapse pruning occurs, and eye closure leads to the retention of more synapses receiving input from the stronger eye compared to the weaker one. This process of synaptic sculpting provides valuable insights into developmental plasticity, serving as a crucial model for investigating various developmental disorders.
The unique dynamics of synaptic connectivity between the dominant and non-dominant eyes exemplify the concept of plasticity, where connections from the most frequently used eye are strengthened, while those from the less used eye are relatively weakened. This model offers a comprehensive understanding of the intricate mechanisms underlying visual development and plasticity.
Exploring mutant mice lacking PirB function while closing one eye revealed unexpected findings of enhanced plasticity, and prompted us to explore the mechanisms of altered plasticity. These studies unveiled PirB's role in modulating synaptic strengthening and weakening, offering insights into its function in normal brain development and potential implications in neurological disorders.
Eventually this led us to think that if these molecules are involved in synaptic pruning in mice, they might also be involved in Alzheimer’s disease, where there is too much synapse pruning and loss.
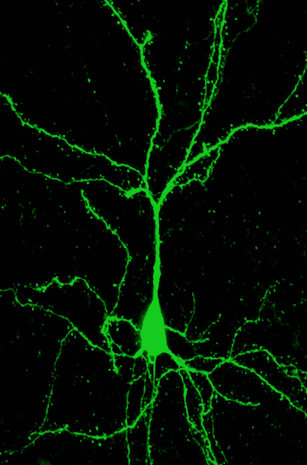
Layer 5 pyramidal neuron - part of the study by Albarran et al Neuron 2021
Could these findings also have implications for other synapse pruning disorders?
Yes they do. If I were to give a talk on this, I might title it "Is Familial Alzheimer's Disease a Developmental Disorder?" This question arises because individuals may carry these mutations from conception, potentially impacting brain development and synapses from the start. Exploring this aspect could offer insights into when clinical interventions might be most effective, but it's a complex puzzle with no clear answers yet.
There are many known genes linked to developmental disorders that also affect spine density, such as fragile X syndrome and MECP2 mutations. Interestingly, while Fragile X syndrome increases spine density, the mice are cognitively impaired. In contrast, in PirB mutant mice, dendritic spine density and excitatory synapses are also increased but the mice seem to be smarter than usual. Upon closer examination, the differences in spine characteristics become apparent.
In PirB mice, cognitive enhancement accompanies increased spine density along the dendrites of cortical and hippocampal pyramidal neurons, with synapses maturing and spines becoming more stable. This diverse array of spine types is crucial for learning and memory, as the mushroom spine type allows for the storage of a wealth of information. Conversely, in fragile X mice, spines are unstable and do not mature, and so the mice lack what is necessary for effective cognitive function.
These distinctions highlight the intricate interplay between different genes and their effects on brain development. Simply observing an increase in spine density doesn't paint the whole picture; careful examination of spine and functional synapse characteristics is essential for understanding the unique phenotypes associated with each mutation.
In addition to your research, you are also the Director of Bio-X, Stanford University’s pioneering interdisciplinary biosciences program. Please can you tell us more about the mission of Bio-X? What advice would you give to those looking to foster interdisciplinary research?
The mission of Bio-X is to facilitate interdisciplinary collaboration in research, aiming to uncover new fundamental mechanisms relevant to human health and disease. As we celebrate our 25th anniversary this year, it's incredible to reflect on how Bio-X has built a community of collaborative faculty and students eager to tackle complex problems using a diverse range of tools, and even creating new ones when necessary.
When Bio-X was named, the intention was clear: biology plus "X" encompassed all related fields, including engineering, chemistry, physics, biophysics, genetics, medical and clinical sciences, as well as even law and business. This expansive approach has enabled us to reach out to faculty across the university, fostering collaborations that bring together diverse expertise to solve pressing issues.
Our website showcases numerous examples of successful collaborations and serves as a platform to communicate our mission. Over the years, we've provided support for faculty and student collaborations through funding opportunities. Currently, over a thousand faculty members belong to BioX, allowing them to apply for seed grants and students to pursue interdisciplinary research projects through Ph.D. fellowships.
While it's challenging to accommodate all proposals from Stanford faculty due to resource constraints, we strive to support approximately 15 to 20% of submissions, all of which demonstrate novel ideas and high quality. This support has not only facilitated groundbreaking research but has also nurtured a vibrant community of collaborators who value the ethos of sharing and cooperation. There are many institutions that have programs like Bio-X, but I don’t think they started as early as Stanford, in 1998!
Undoubtedly, the COVID-19 pandemic has affected our activities, but as we gradually return to in-person events like symposia and gatherings in the cafeteria, we're reminded of the importance of personal interactions in fostering collaboration. After all, resources such as research funding and food can be powerful catalysts for bringing people together and sparking new ideas.
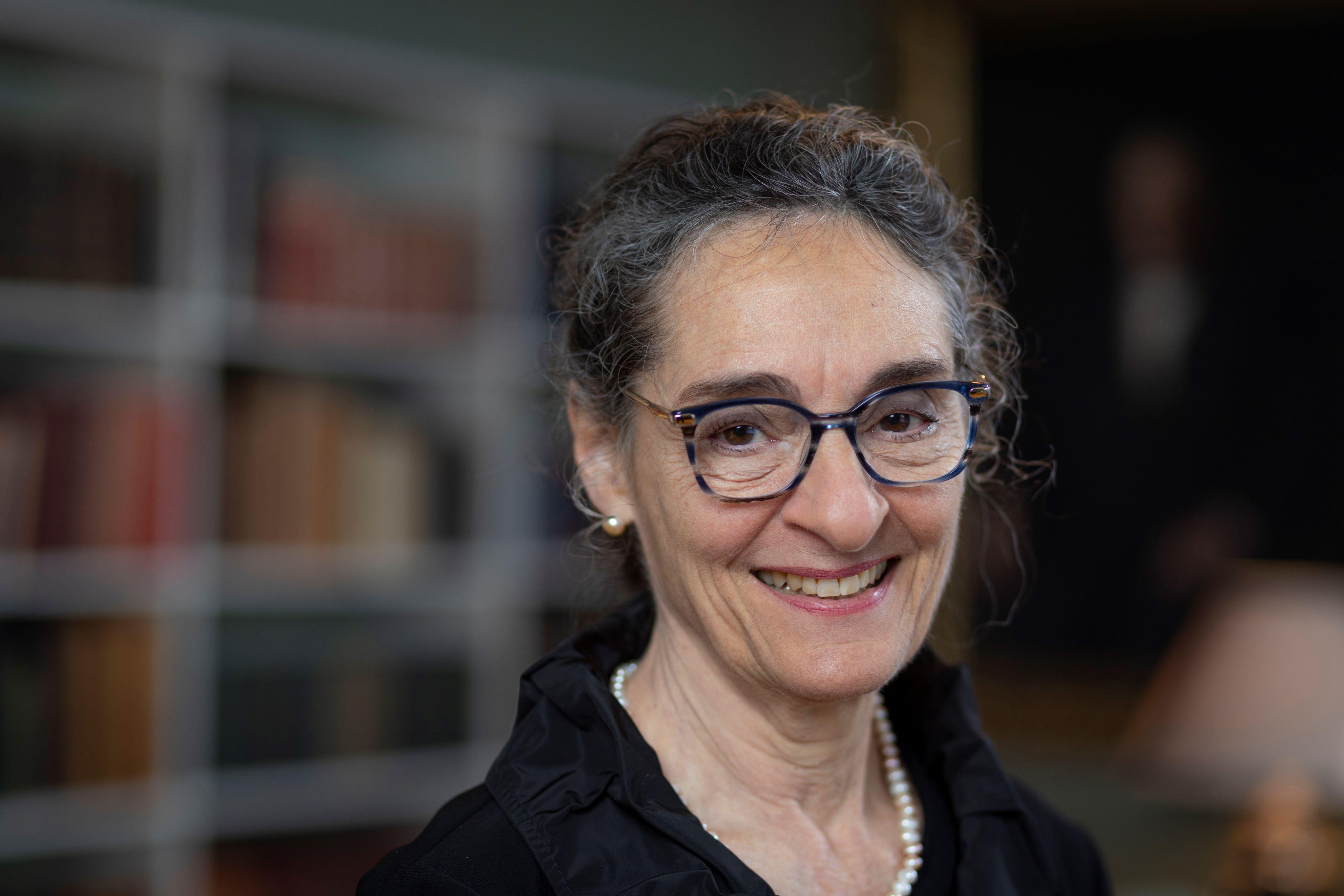
About Professor Shatz
Carla J. Shatz is Sapp Family Provostial Professor of Biology and Neurobiology and the Catherine Holman Johnson Director of Bio-X, Stanford University’s pioneering interdisciplinary biosciences program. She received her B.A. in Chemistry from Radcliffe College in 1969, an M.Phil. (Physiology; 1971) from University College London as a Marshall Scholar, and a Ph.D. (Neurobiology; 1976) from Harvard Medical School. Shatz joined the faculty at Stanford in 1978, then moved to University of California at Berkeley in 1992, and to Harvard Medical School in 2000 as the first woman Chair of the Department of Neurobiology. She returned to Stanford in 2007 to direct Bio-X. Dr. Shatz is a neuroscientist who has devoted her career to understanding the dynamic interplay between genes and environment that shapes brain circuits - the very essence of our being. Shatz has earned many honors and awards, including election to the National Academy of Sciences, the American Philosophical Society and the Royal Society of London. She received the Gruber Neuroscience Prize in 2015. In 2016, she was the recipient of the Champalimaud Vision Prize, and also the Kavli Prize in Neuroscience for the discovery of mechanisms that allow experience and neural activity to remodel brain circuits. In 2018, she received the Harvey Prize in Science and Technology from the Technion Israel Institute of Technology.