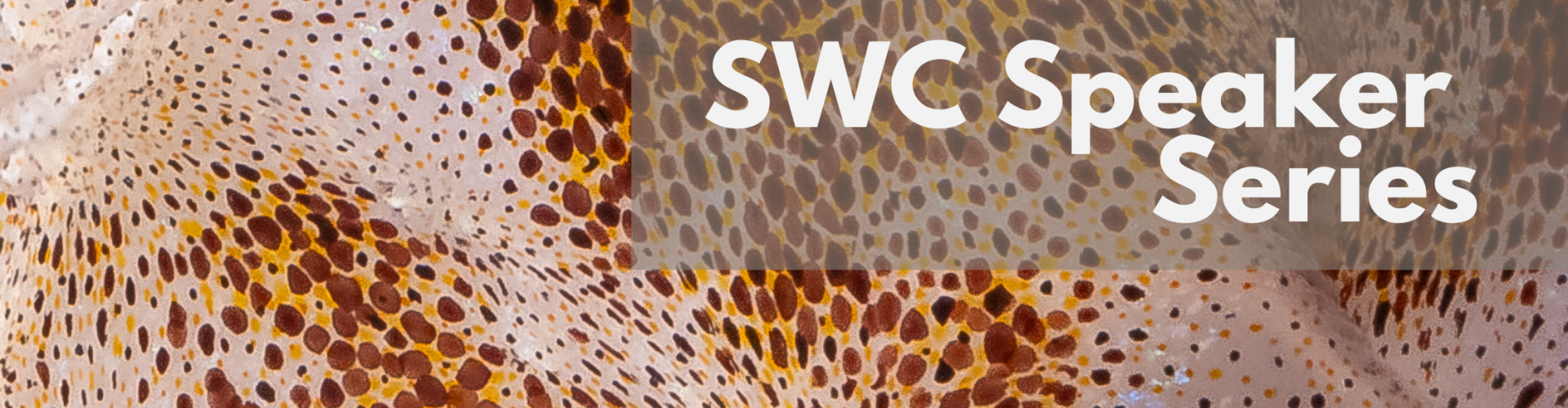
Unveiling camouflage and decoding neural mechanisms in cuttlefish
An interview with Dr Tessa Montague, Columbia University, conducted by Hyewon Kim
How could the display technology used in reading tablet devices be used to study how camouflage takes place in the skin of cephalopods like cuttlefish? In this Q&A Dr Tessa Montague, the last 2023/24 ENSS speaker, shares insights into her fascination with cuttlefish, the challenges and triumphs of studying them, and the innovative methods employed to understand their camouflage behaviour and neural mechanisms.
What prompted you to apply for the SWC Emerging Neuroscientists Seminar Series (ENSS)?
I've been watching the evolution of the Sainsbury Wellcome Centre for a while as I’m from London, and was excited by the creation of a cutting edge neuroscience institute, but it didn’t exist when I left for the U.S. to pursue my PhD. I’d been waiting to have enough data to give the talk, so I finally applied this year. It was funny that three of us postdocs were chosen from the same institute! I guess that can happen when the candidates are chosen blind.
How useful has the experience been?
It's been wonderful. It's exciting presenting my work to different audiences, and the questions I received today were really thoughtful. I often get asked quite similar questions, but today I got a lot of new ones, which was fun to think about. Meeting all the different scientists, hearing about their work, and finding connections between their studies and how they've influenced each other is a good sign of community. Overall, it was a fun experience.
What advice would you give to those considering applying to ENSS 2024/25?
I was worried that I wouldn't have enough of a developed story to apply to the program, and that most people apply to ENSS when they're already on the job market. But I don’t think you have to have a complete story. Sometimes it's actually fun for people to see a work in progress because then they can follow how it develops. I like finding out which parts of the project people find particularly interesting or challenging. If you have a finished study, perhaps you don't get that kind of feedback.
Why did you choose to study cuttlefish? Are they commonly used model organisms in neuroscience?
For my PhD, I studied embryonic development in zebrafish, one of the common model organisms in biology. I really enjoyed what I was doing, but in terms of the questions I was asking it felt like a crowded field, and I got a little nervous about my future. A lot of the talks that got me the most excited and inspired by science were by people working on big questions in new model organisms. When I imagined studying something like that, I got a feeling of excitement rather than fear. So, I decided that that was the direction I was going to head in for my postdoc and probably for the rest of my career; I just had to find the system and the question.
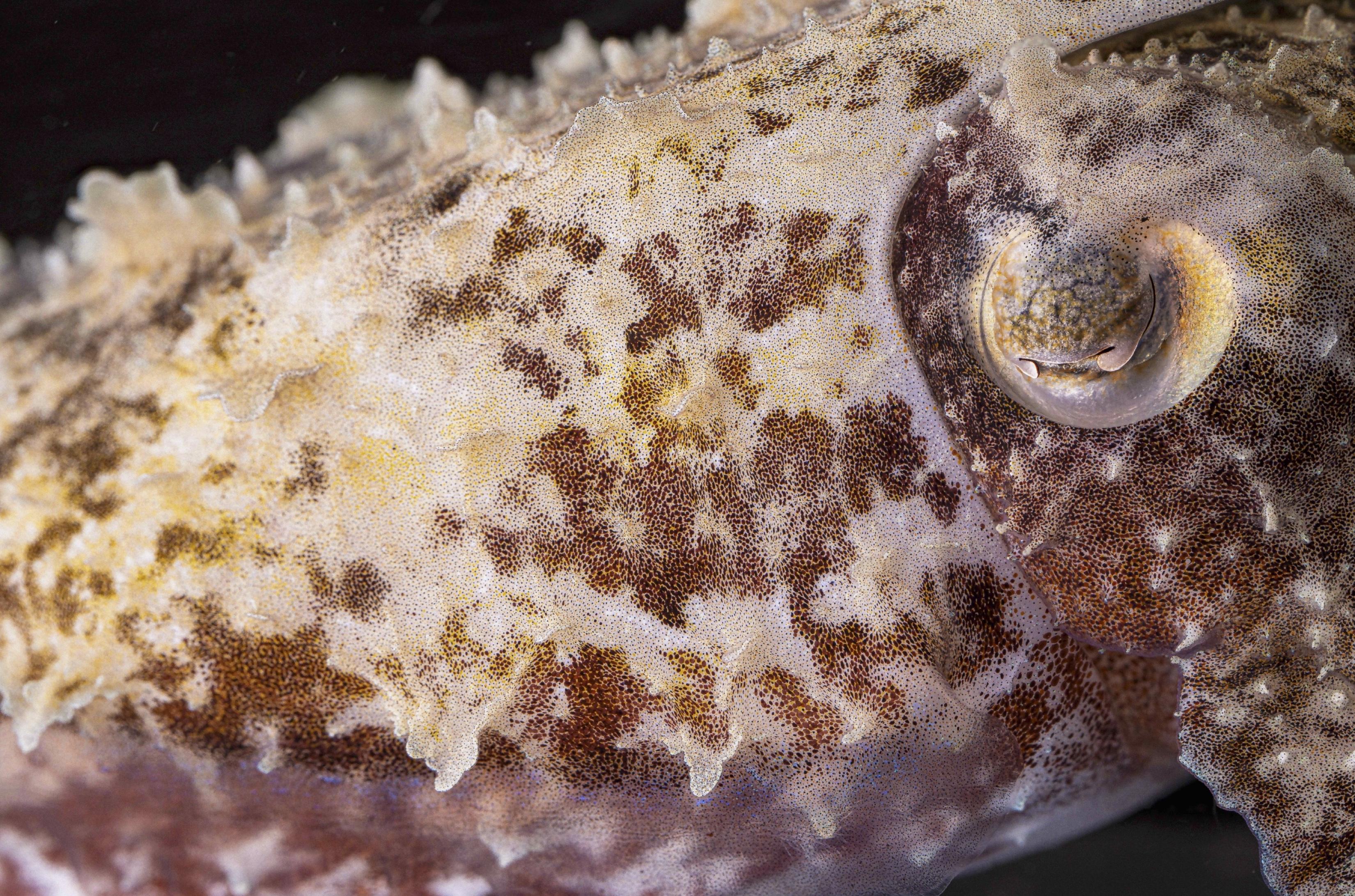
I took a 6-week embryology course at the Marine Biological Laboratory (MBL) in Woods Hole, in which we were introduced to around 100 organisms from across the evolutionary tree. As I explored each of them I would ask: ‘Is this my future? Is that my future?’ In the final week, we had a 10-minute talk from Bret Grasse, who was the MBL’s new Manager of Cephalopod Operations. In his short talk, he introduced cephalopod biology and showed a few videos of their incredible behaviours. I felt like my whole life flashed before me – this was what I had been looking for.
In the end, even though this project started from a sense of fear, in the end I guess it took some guts because I had to start from scratch. There were basically no tools or almost anything known about the particular species that I wanted to work on. We had to build an animal facility in the Axel lab from nothing and figure out the most basic things to eventually earn the opportunity to move onto the really interesting questions.
How was it initially discovered that camouflage in cuttlefish is achieved by motor neurons in the brain projecting to chromatophores in their skin?
There is a rich history of studying the cephalopod brain, particularly in the 20th century. A number of scientists like J.Z. Young and Brian Boycott studied cephalopods with limited tools, yet made really important insights. They carefully dissected the animals, sliced their brains, stained neurons and traced them to see where they project. They created a map of the cephalopod brain and discovered that neurons project all the way to the skin to control colour change. They also severed the nerves that control the skin and the animals went pale – the "lights went off”.
As far back as 300 BC Aristotle wrote about the remarkable behaviour of cephalopods, so these animals have captured the imagination of scientists for thousands of years. Only in the last couple of years have we had the tools, or the potential to create the tools, to uncover the neural basis of their behaviours.
Why investigate the neural basis of camouflage?
For some reason, I didn't know about cephalopod camouflage until only a few years ago. Probably many kids learn about camouflage visiting public aquariums or reading about it in books, but somehow those books got past me. When I actually saw dynamic camouflage as an adult, I thought it was magic – I couldn't believe my eyes. The behaviour gripped me as a truly fascinating process.
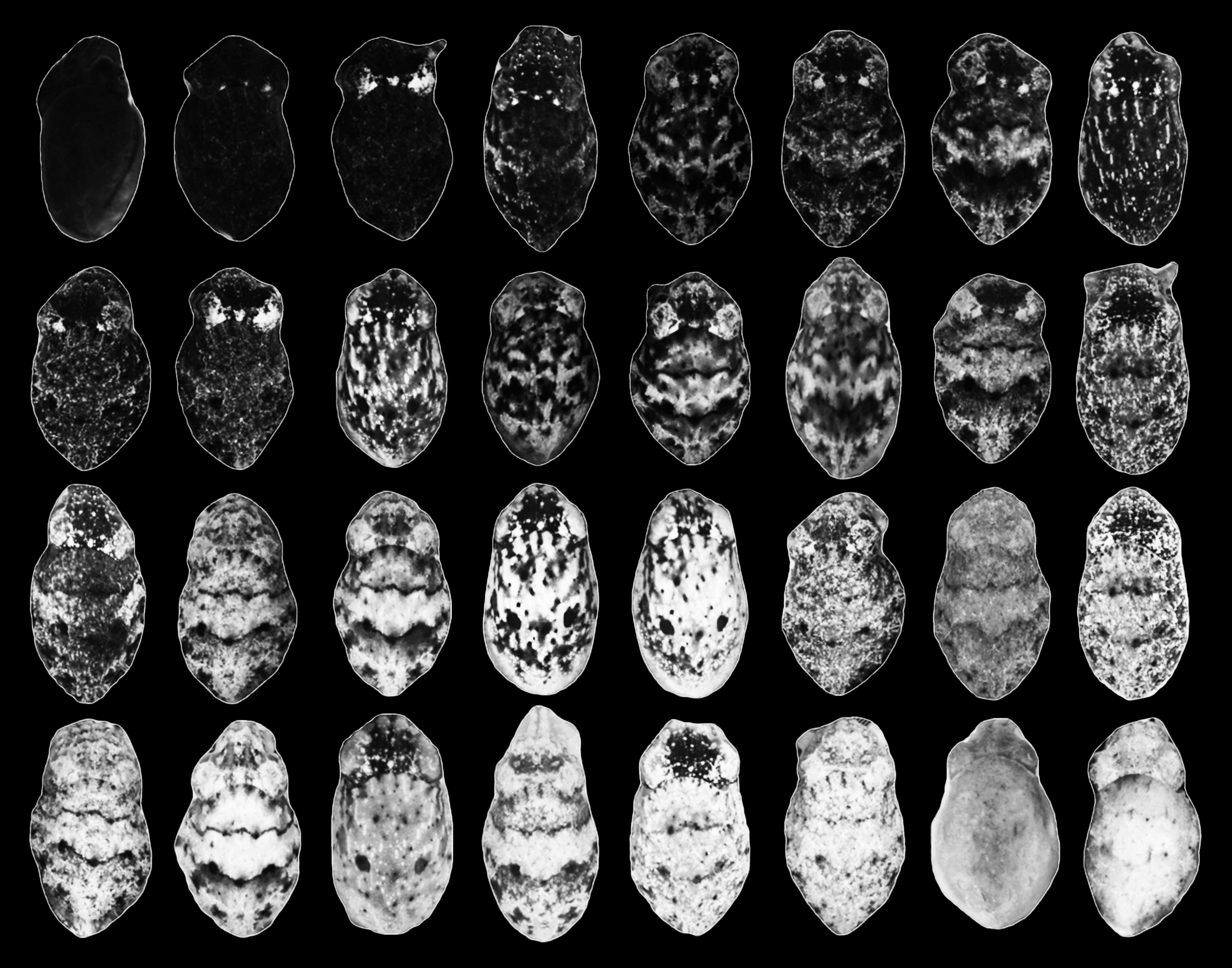
But I wanted to ask a fundamental question beyond what this particular marine creature does: what is the principle about the brain that we can uncover by studying this? I reasoned that if an animal is camouflaging, it's basically showing you what it sees on its skin. Therefore, it could teach us about the inner workings of the brain.
What was the motivation for developing the dwarf cuttlefish?
In my career so far, I've studied almost every classic model organism – fruit flies, mice, yeast, chicks, zebrafish. So I was very aware of the type of toolkit you need to study biological mechanism. If I was going to study a new system, I wanted genetic tools so I needed a species where that would be feasible. One of the reasons I got so excited by Bret’s talk at the MBL was that he and others had already developed methods to breed these animals in the lab. Of the millions of animal species in the world, only a fraction of them can be bred under lab conditions. There's so little we know about what makes animals comfortable, want to mate and how to get embryos to develop to term. The fact that Bret had already cracked that problem really inspired me to feel like we could do it. We have an amazing cephalopod facility manager at Columbia, Connor Gibbons, who runs our facility. His incredible expertise with the animals makes all of our experiments possible. Our animals readily mate, lay loads of embryos and perform incredible behaviours before our eyes every day.
Could you describe your visually-evoked camouflage behavioural paradigm?
We know from looking at animals in the wild that they camouflage very well to three-dimensional rocks, corals, and seaweed. But if we wanted to get a neural handle on what's happening in the brain, we needed to carefully control the stimuli presented to the animal: ideally we would change the stimulus on a second or millisecond timeframe, perhaps with a digital tank. The challenge was that regular TV screens don't seem to work with cephalopods.
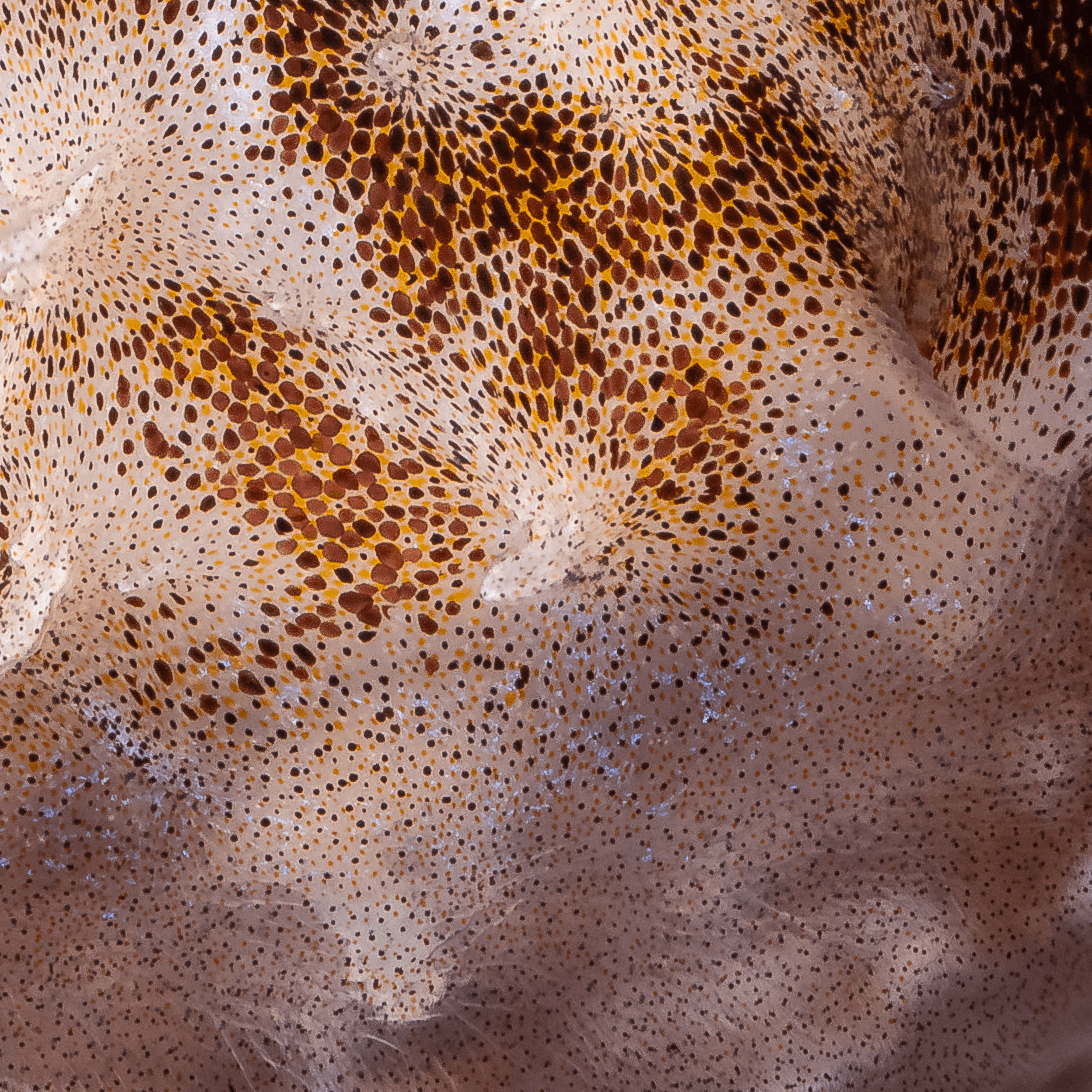
We ended up using a technology called E ink, which is the technology used in Kindles. It uses pigmented particles that have an electrical charge to create different patterns. And though they don't refresh as quickly as a TV screen, they do so fast enough for us to be able to change the environment around the animals in less than a second. And rather than emit light, which is not very naturalistic for an underwater animal, they just reflect light so we can use overhead lighting that’s more like the sun.
How do you currently hypothesise visual information is deconstructed in the brain to be reconstructed into an image of the physical world on the skin?
The short answer is we don't know, but we have some hypotheses about how it might work. This is particularly the work of Erica Shook, a graduate student in the Axel lab who’s co-mentored by Larry Abbott. Her idea is that cuttlefish live in environments rich in visual textures—homogeneous images with repeating elements and random variation like sand, seaweed, rocks, and corals. Rather than represent every single pixel of their environment in the brain, which is inefficient, cuttlefish might represent visual textures in a low-dimensional form, using a set of statistics. They could compress the visual world into a set of statistics (which is pretty efficient), then decompress the statistical representation into an approximation of the world on the skin. Interestingly, it’s precisely because our brains represent textures in a summarised way that animal camouflage tricks us—they don’t have to perfectly match their environment to evade our detection.
Could you talk about the change in pigment during aggression in cephalopods? Was paying attention to that behaviour a natural byproduct of studying the neural basis of camouflage?
One of the interesting things about cephalopods is they also use their neurally-controlled colour change to communicate with other animals through a series of social patterns. As you suggested, at first, we were ignoring the aggression-invoked pigment changes in their skin. But as time went on, we realised that the innate skin patterns were very stereotyped compared to the camouflage patterns. They’re also quite robust: if we have an animal that, for some other reason, is distracted or irritated, or there's something going on that prevents it from camouflaging, if we present the right social stimulus, it immediately flares into an aggression pattern.
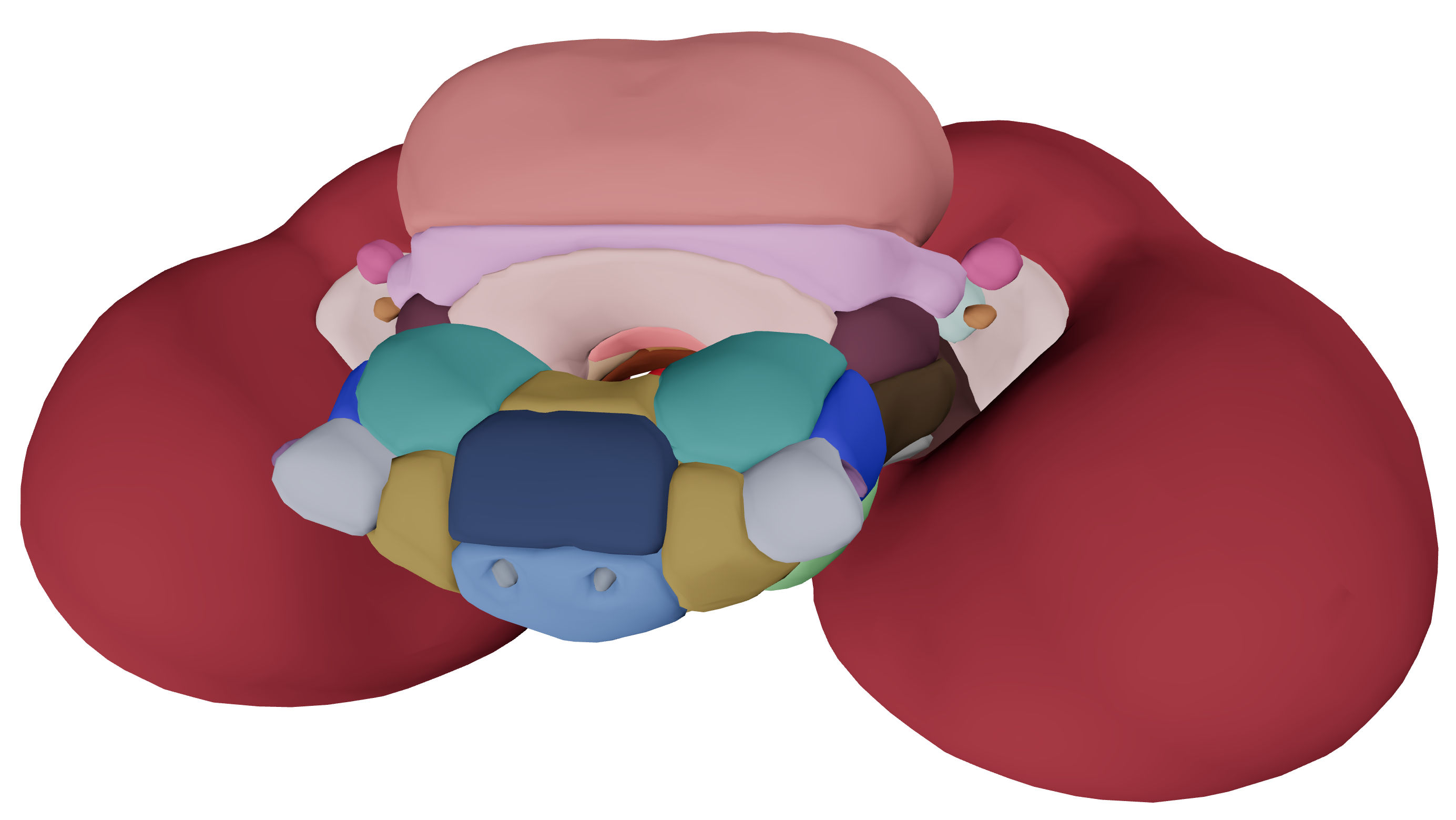
We're planning to study the aggression behaviour in its own right because we think these social skin patterns are broadcasting the animal's internal state. It's another way that the animal shows us what it's thinking. But also, the skin pattern is a tool for us. By studying a stereotype pattern, we can look inside the brain and see how that’s represented as a comparison to the more flexible camouflage patterns.
What are some of the next steps that you’re excited about?
I think we're finally close to having a transgenic animal that expresses a calcium indicator in the brain. This has been the biggest challenge and goal for the entire project, but I think we're finally close. That will allow us to image neural activity during camouflage to uncover how the visual world is represented and transformed in the brain.
You’ve partaken in projects to bring science education and research beyond the lab. Can you tell me more about how you created an undergraduate neuroscience course in a woman’s prison?
Taking science to different environments and communities is something I've always been passionate about. I've actually wanted to teach in a prison for a long time. Finally, the opportunity arose when I found out about an organisation called Hudson Link that collaborates with Columbia University and some other institutions to allow incarcerated people to earn degrees.
I teamed up with another postdoc, Shai Berman. The real challenge was that no technology is allowed in prison. We couldn't use PowerPoint, we couldn't access the internet, and we didn't have phones or internet access while we were there. All we had was a whiteboard and paper.
Another challenge was that we had to submit all of our teaching materials three months in advance to get approved to enter the prison. It was difficult because we had to create a course from scratch. Neither of us had created a neuroscience course before, and we had to guess what the right level would be, and then nothing could be changed along the way.
But it was really enjoyable in the end. The students were fantastic. They were so engaged and derived so much joy from learning, which can be difficult to find in a typical classroom. It was challenging to teach on a whiteboard, but it was also very useful because we got down to the basics.
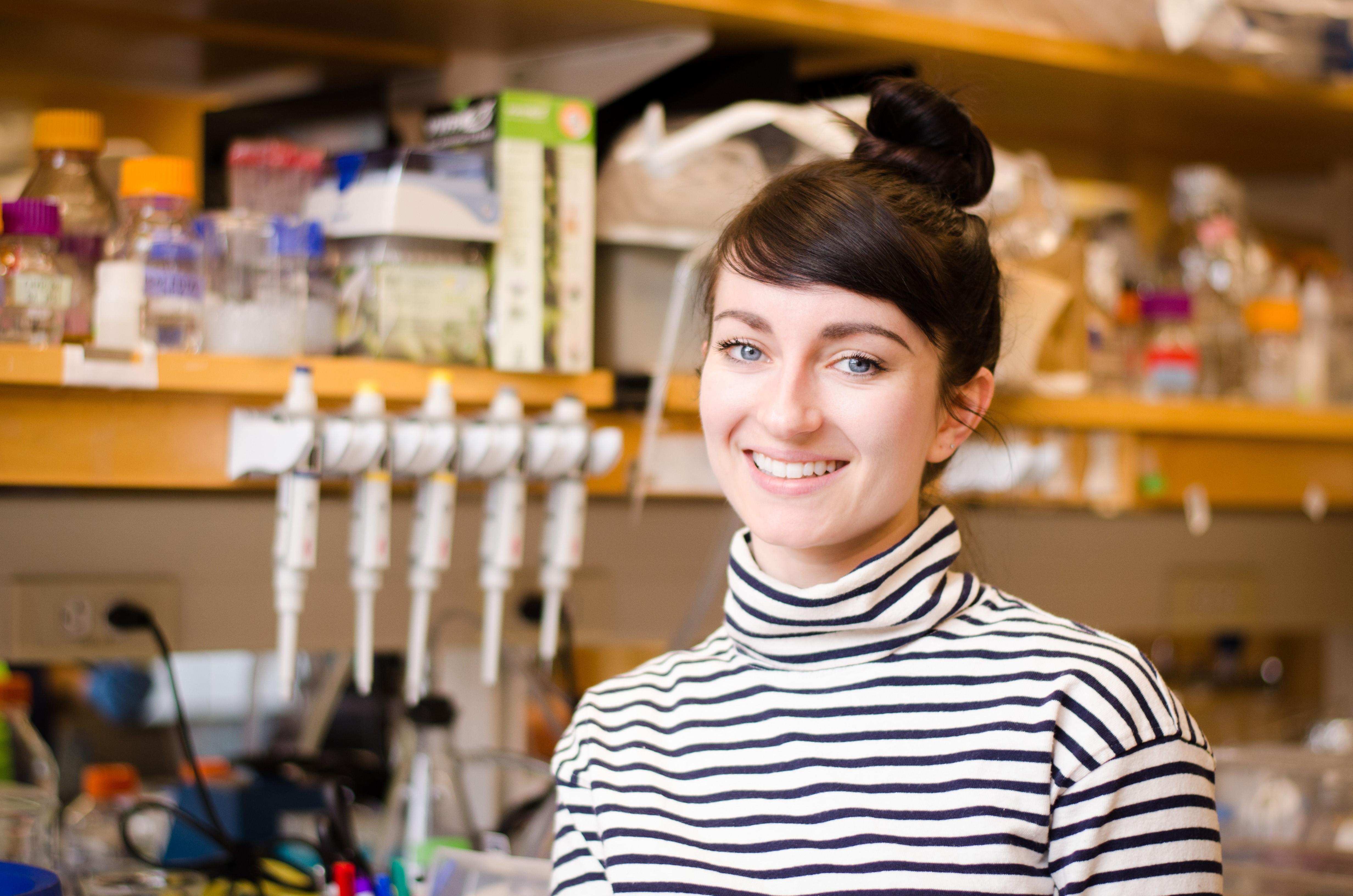
About Dr Montague
Tessa Montague is a postdoctoral fellow and HHMI Hanna Gray Fellow in the Axel lab at Columbia University. Tessa completed a B.A. in Natural Sciences and an M.Phil. in genetics at the University of Cambridge, and a Ph.D. in biochemistry at Harvard University, where she worked in the laboratory of Alex Schier. As a graduate student, Tessa dissected molecular pathways underlying zebrafish embryogenesis and co-created the CRISPR web tool, CHOPCHOP. In the final year of her Ph.D., Tessa attended the Embryology Course at the Marine Biological Laboratory in Woods Hole, where she became fascinated by cephalopods (cuttlefish, octopus and squid). This inspired her to return to Woods Hole as a Grass Fellow, and to launch her postdoctoral research program on cuttlefish camouflage. Tessa is passionate about bringing science education and research beyond the lab. Among other projects, she recently co-created and taught an undergraduate neuroscience course in a women's prison.