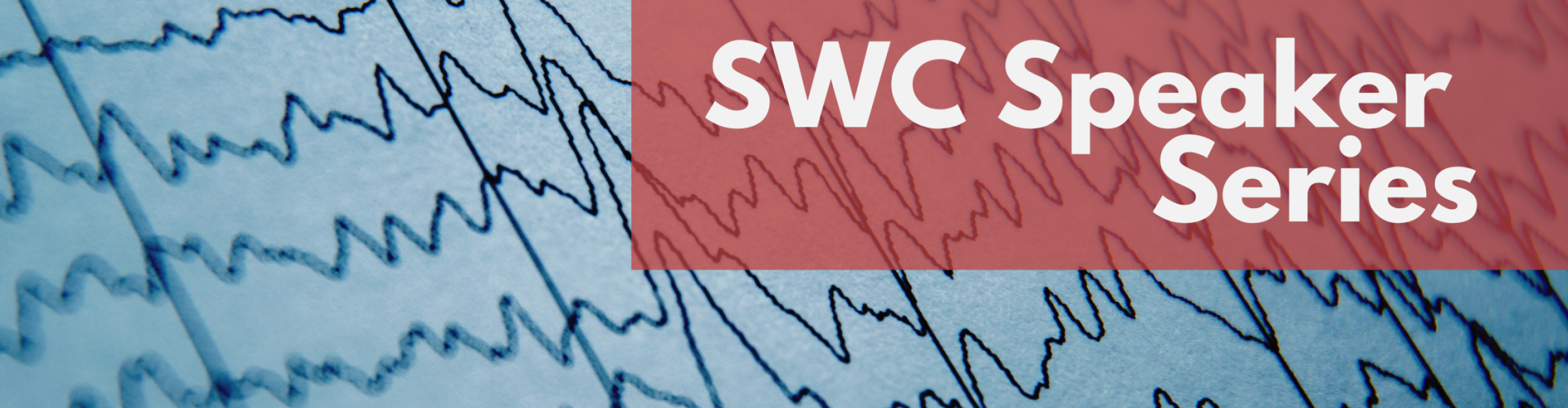
Brain waves: causes, correlations and controversies
An interview with Dr Vikaas Sohal, University of California, San Francisco, conducted by April Cashin-Garbutt
Rhythmic brain waves, or neural oscillations, were first observed over a century ago, but their functions still remain controversial. While we know that certain brain waves correlate with different mental activities, there are many unanswered questions. Are these oscillations a mechanism that underlies brain function, or merely a byproduct of brain activity? How do they influence behaviour? And what is the importance of synchrony across different brain regions?
These are some of the questions that drive Dr Vikaas Sohal’s work. Based at the University of California, San Francisco, his lab investigates the brain circuits underlying fundamental aspects of cognition and emotion. Dr Sohal’s team have shown that deficits in gamma wave synchrony and the neurons that give rise to it might contribute to cognitive issues at the core of schizophrenia and Alzheimer’s disease. In a recent SWC seminar, he spoke about his latest work, including finding that gamma waves play a key role in cognitive flexibility – specifically the brain’s ability to learn new rules.
In this Q&A, Dr Sohal shares his key findings, clinical implications and next steps.
How common are synchronised oscillations in neural systems, and why has their functional significance long been controversial?
People would agree that these oscillations are very common. When you look at an electroencephalogram (EEG), you see these wiggles - sometimes fast, sometimes slow. In the early the days of EEG people named them; alpha, beta, delta, gamma waves, depending on their frequency.
We know that spikes in electrical activity in neurons have functional significance, as that’s how one neuron excites or inhibits another by releasing neurotransmitters. However, for oscillations, people just observed them, so it wasn’t clear if they had functional significance. Just observing them doesn’t mean they do anything; they could be explained as a byproduct of other things happening in the nervous system. Our inability, until recently, to do experiments that directly test their significance led to this debate.
But I think another reason for the controversy is that it is natural for scientists, when we see something, to imagine an explanation or purpose for it. And it is also natural when one scientist suggests something for others to be sceptical – so there is a lot of that too.
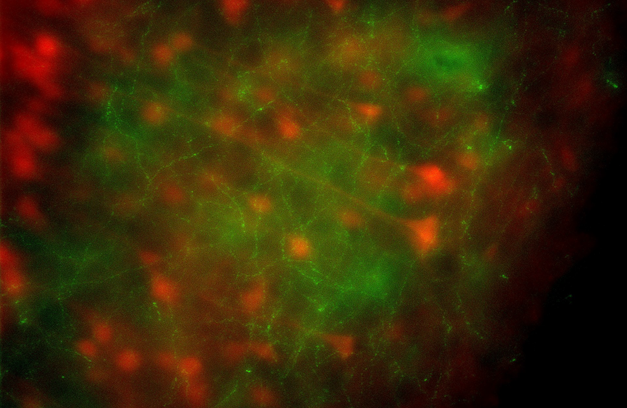
Could you elaborate on how your lab is uncovering the functional role of synchronisation in the brain?
We have been taking advantage of two recently developed tools that are afforded to us by modern neuroscience. One is, of course, optogenetics.
This technique is still amazing to me. I was part of Karl Deisseroth’s lab when he was developing the technology 20 years ago. I consider myself very fortunate to have been in the right place at the right time.
On the 10th anniversary of optogenetics, Nature Neuroscience had a special issue about the technique, and they published some of the original comments from the reviewers at the time. Some of the reviewers were very sceptical that it would ever be useful for anything. I didn't immediately grasp how transformative it would become either.
The task of getting at the causal role of electrical activity patterns of neurons is possible with optogenetics. What's really key about optogenetics is fast temporal precision - we can turn on neurons with millisecond accuracy. And that allows us to control if the oscillations of two groups of neurons are going to be in or out phase with each other. We can compare how stimulation in phase or out of phase might lead to different effects on behaviour. The precision is essential.
The second technique that has been very useful in our work has been using genetically encoded voltage indicators. Just like radio signals or wireless signals propagate passively through the air, electrical signals propagate passively through the brain. This means if you pick up an electrical signal in region A and region B, it is very hard to say that A and B are oscillating and are synchronised.
Using genetically encoded voltage indicators we can measure signals that we know are coming from specific cells, and cell types. That makes it possible to precisely pull out signals which otherwise might be lost in a mix of electrical signals originating from different places and even from different cells in those different places. Using genetically encoded voltage indicators has really been transformative for us.
Were you surprised to find that gamma wave synchrony plays a critical role in cognitive flexibility?
I have been surprised at how specific the role is. When I first was starting this work, I thought that gamma oscillations might be important in general. But what we’ve found is that they seem to be recruited at very specific moments for very specific purposes.
In our case it seems to be that gamma oscillations are recruited and are important for updating something in the brain, so that you pay attention to something new, and use that information to make decisions.
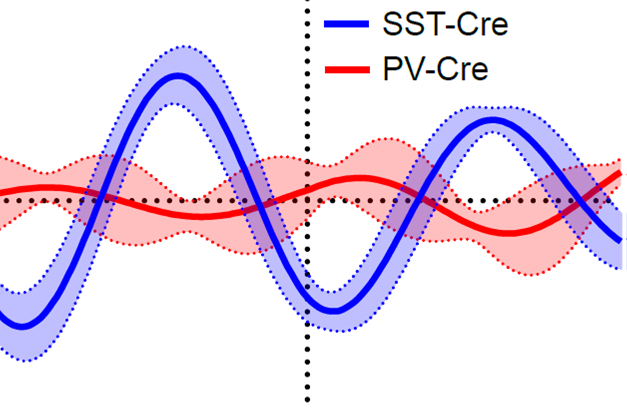
How are you exploring where this synchrony comes from?
It is a little bit of a game of whack-a-mole in neuroscience. When you discover something, then you ask, well, where does that come from? So we have been exploring different ideas about the origins of gamma wave synchrony.
One idea is that gamma wave synchronicity might be caused when cells in other brain regions contact parvalbumin interneurons in the prefrontal cortex and excite them. This may then cause in increasing gamma oscillations or increasing gamma synchrony.
Another idea is that neuromodulatory input to the prefrontal cortex plays an important role. We particularly interested in dopamine as a neuromodulator.
And then the third idea we have is that when there is new or unexpected information, it causes these brain regions to work together to generate the gamma oscillations. Gamma oscillations could be an emergent property of networks when they receive conflicting or unexpected information. That’s going to be very hard to test, I’m not yet sure how we would do that. We’ll test that one last!
Why are you focusing on the neural synchrony in relation to cognition and emotion?
In relation to cognition, it is because in schizophrenia the most robust finding in postmortem brain tissue is that parvalbumin interneurons have abnormalities.
A lot of research, including my own, suggested that parvalbumin interneurons play key roles in gamma oscillations. We want to really understand why gamma oscillations and the synchronisation of those oscillations are so important that disruption contributes to conditions like schizophrenia.
We are interested in emotion because in EEG recordings of humans in different emotional states, you can pick up these oscillations. Our hypothesis was that something like mood or emotion is unlikely to be encoded strictly at the level of one brain region. Instead, we think this would be encoded at the level of networks and interactions between brain regions. To capture that we looked at the synchronisation of oscillations between brain regions.
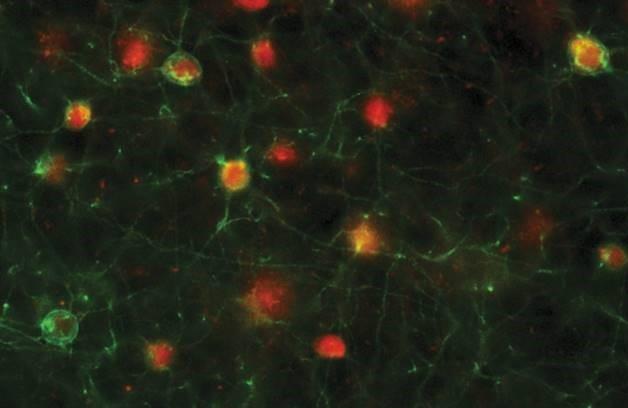
What are the potential clinical implications? Could synchronised oscillations be going awry in some conditions?
The condition that this might be most relevant to is schizophrenia, where we know there are deficits in gamma oscillations, and there are deficits in cognitive flexibility.
Alzheimer’s is another condition where we know that the parvalbumin interneurons are broken. We know that the gamma oscillations are deficient, there is intriguing evidence that targeting gamma oscillations in unconventional ways may lead to improvement of the plaques in the brain that are present in the disease. This is very much an active area of research.
I think in autism there is a lot of evidence that parvalbumin interneurons are dysfunctional. It could be that problems with gamma oscillations lead to some of the rigid behaviours that are hallmarks of autism. I think that remains to be worked out as well.
I’d add that rigid thinking and inflexible behaviour also occur in conditions such as depression and OCD, and so we wonder if some of these results might be relevant there, too.
What are the most significant implications of your research on neural synchrony for the broader field of neuroscience?
We have made a huge amount of progress on the ‘what’ and ‘which’ questions. Such as; what brain regions, and which neurons in those brain regions, are involved in certain behaviours?
I think we have made less progress on the ‘how’ questions. How do these neurons perform certain computations? Why is it that at certain times their activity changes in certain ways?
I think that identifying synchrony may identify brain states that are conducive to certain computations, and changes in the way that neurons encode information.
We know that neurons are connected to each other, and neurons encode these variables. So one question is how do we build a model for how that actually emerges from these connections. Thinking about oscillations will be important for doing that.
What is the next piece of the puzzle that you are going to focus on?
We are trying to just keep digging down on the controversial question of whether oscillations are functional, or a byproduct of something else happening in the brain.
The way you resolve that controversy is by showing mechanistically how these oscillations work. I think we have shown they are important for certain behaviours. We've started to show why, but we need to go deeper.
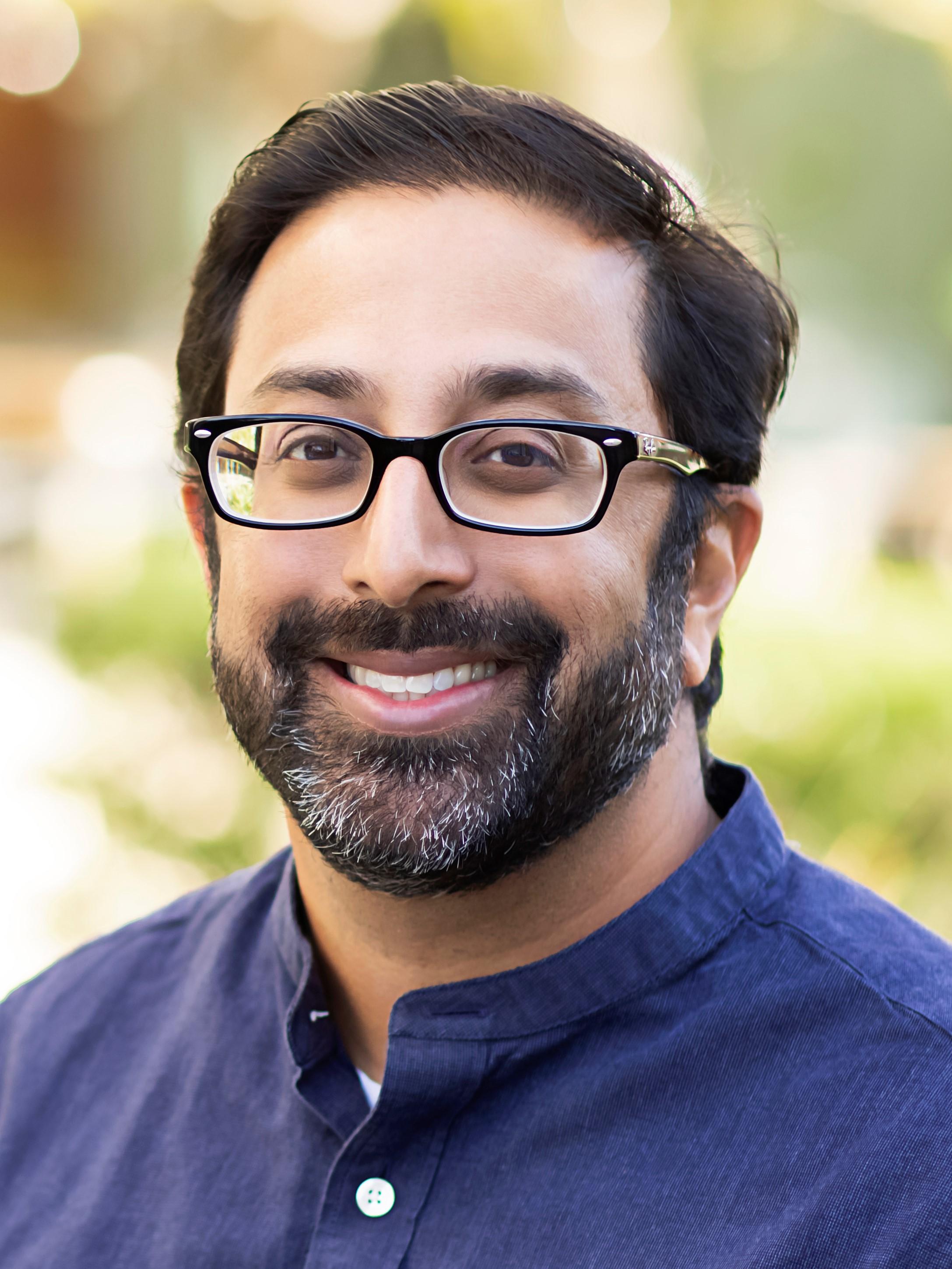
About Dr Sohal
Vikaas Sohal studied Applied Mathematics at Harvard and Cambridge before completing his MD and PhD degrees at Stanford. During graduate school he studied thalamocortical oscillations with John Huguenard. He stayed at Stanford for psychiatry residency. During that time, he worked with Karl Deisseroth, carrying out some of the first experiments using optogenetics. In 2010, he started his independent laboratory at UCSF which studies how emergent patterns of cortical circuit activity contribute to cognition and emotion. Dr. Sohal has received several awards for his research including the NIH Director’s New Innovator award, the A.E. Bennett Research award from the Society of Biological Psychiatry, the Daniel Efron award from the American College of Neuropsychopharmacology, and the McKnight Memory and Cognitive Disorders award. He is a board-certified psychiatrist and continues to see outpatients in the Early Psychosis clinic at UCSF.