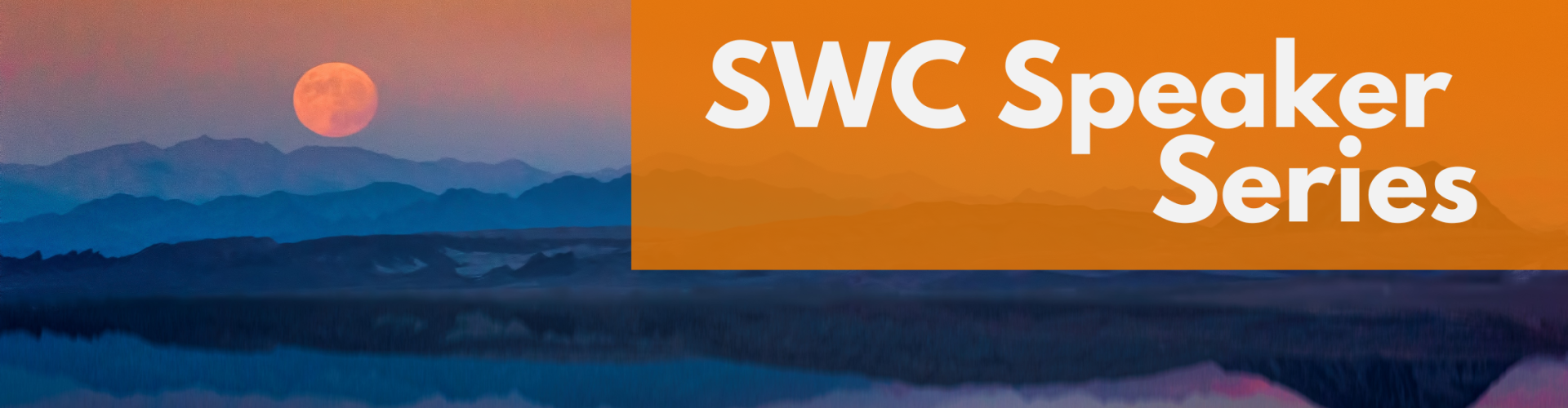
From dawn to dusk: the science behind light and circadian rhythms
An interview with Professor Stuart Peirson, University of Oxford, conducted by April Cashin-Garbutt.
The impact of blue light has been a hot topic in the media, but how much should we really worry about its effect on our circadian rhythm? This was one of the questions I was eager to ask Stuart Peirson, Professor of Circadian Neuroscience at the University of Oxford, after his thought-provoking seminar at SWC.
Professor Peirson’s journey into the world of circadian rhythms began serendipitously when his PhD supervisor, fresh from a trip to New York, suggested he explore the pineal organ. The pineal gland is where melatonin is produced, which plays a crucial role in the regulation of circadian rhythms. This unexpected detour set the stage for his future research.
In this Q&A, Professor Peirson shares key findings from his research on the regulation of circadian rhythms and sleep in mammals, discussing implications ranging from jet lag to the design of lighting in the buildings where we spend most of our time.
Do all organisms possess a circadian clock and how much do they vary between species?
Virtually every organism possesses a circadian clock – from bacteria up to humans. There are some very odd examples such as certain types of cave fish that don’t (but other types that do!)
The specific mechanism can be quite varied. However, a lot of the basis of our understanding of mammalian (including human) circadian rhythms comes from work that was done in fruit flies, drosophila. The 2017 Nobel Prize in Physiology or Medicine was awarded for the basic understanding of the molecular mechanisms of circadian rhythms.
While it has been around 500 million years since humans and flies last shared a common ancestor, our circadian rhythms are based on very similar overall mechanisms. Genes are expressed, translated into a protein product, and those proteins feedback to regulate their own expression over 24 hours.
There are networks of different genes and proteins that interact to form a molecular clock that occurs in cells and tissues throughout the body. The basis of that molecular clock is what is happening in the master clock in the suprachiasmatic nuclei (SCN) in the hypothalamus in the brain. This is what generates the behavioural rhythms that we’re familiar with.
Plants also have the same sort of molecular, transcriptional, translational feedback loop, but the genes are different. However, a lot of it is still based on the same basic mechanism in terms of genes being expressed, producing proteins that inhibit their own production after 24 hours.
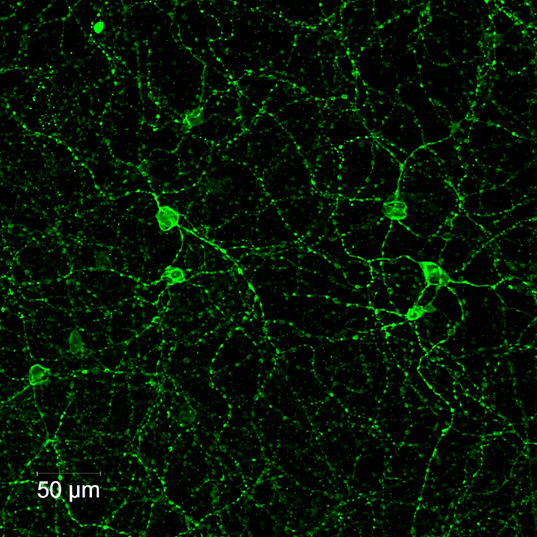
How much is known about the way light regulates circadian rhythms?
There have been huge advances in the last 25 years since the discovery of melanopsin-expressing intrinsically photosensitive retinal ganglion cells. The original studies were done by Russell Foster’s lab where they looked at blind mice with no rods or cones and found that they can still respond to light in terms of circadian rhythms.
This was the basis for subsequent work by other colleagues in the field who discovered that a small percentage of ganglion cells that project to the brain are intrinsically light-sensitive because they express a blue light-sensitive pigment called melanopsin.
Should we worry about the effects of artificial light, and in particular blue light from our devices, on our circadian rhythms and overall health?
Artificial light can be problematic because our environment is full of artificial light these days. Another big factor is that we get very little natural light. Historically, we would have been exposed to nice bright light during the day and no light at night. Whereas now we’re less exposed to light during the day because we’re indoors all day, and then we’re exposed to light on an evening.
Light has a different effect on the clock at different times of day. On an evening, light will delay your circadian clock, so it will start later the next day. At the end of the night, near dawn, light advances the phase.
The start of the night is one of the times when we’re most sensitive to light and that’s when we go home and turn the lights on!
One of the best examples of this was a study published in Current Biology by Ken Wright’s lab in Colorado. They measured people’s light exposure in their normal environments and then they took them camping where they weren’t allowed any artificial light sources. They showed that under the artificial light condition, their melatonin onset, midpoint and offset was all delayed for several hours and their sleep was delayed.
Whereas under normal natural light conditions, melatonin onset and offset is beautifully coupled to the sunset and sunrise. And so, we have a beautiful physiological system that synchronises our rhythms to the natural solar cycle, but the exposure to artificial lights leads to delays.
There has also been a lot of work on artificial light at night both in animal models and humans showing that there seem to be harmful effects which range across many different aspects of physiology. For example, there is work from the UK Biobank showing mood was associated with light exposure. Research in animal models has also shown disruption of mood, learning and memory, immune function, endocrine function and many other processes.
Of course, no one is going to go back to living with only natural light, but we can at least design lighting better so that it takes our circadian function into account. The lighting industry are now getting interested in “human-centric lighting” and the WELL Building guidelines take lighting into account – including the non-visual aspects of light. This is a great example of a basic neuroscience discovery that was made in 2002 that is being used in building design around 20 years later.
Many people have heard about the effects of blue light on sleep and the circadian rhythm, but there is a bit of a misrepresentation in terms of the circadian effects of light. Melanopsin is more sensitive to blue light as it has a peak sensitivity around 480 nm (blue/green) and under lab conditions, this is what humans are most sensitive to. But, the absorption of these pigments is like a bell-shaped normal distribution, so while the peak is at 480 nm, melanopsin can also absorb red light, it just doesn’t do it very well.
If you look at these responses to light, they look like a s-shaped drug-dose response curve with the dose of light on the x-axis and the biological response on the y-axis. However, the x-axis is on a log-scale. This means that if you block the effects of blue light, you reduce the biological response by shifting to the left on the x-axis, but because it is a log scale, you usually don’t shift very far!
Also, because it’s like a drug-dose response curve, you go from no effect to having an effect, to plateau where you won’t get any more effect. So, if the light’s very bright, blocking blue light won’t do anything as you’re already saturated. If it’s very dim, the light is not doing anything to begin with so blocking blue light won’t have any effect. Only in that middle phase, where it is changing exponentially, will blocking blue light have an effect. But it is still on a log scale, so you’re only moving a small amount.
In comparison, the visual system works over a billion-fold range between dim starlight to a bright summer’s day. This means our visual system is quite tolerant of changes in intensity. For example, we can drop our light intensity by ten-fold and our visual system still works quite well.
The bottom line is that yes blue light technically has more effects that other wavelengths, but actually it depends on the intensity and just reducing the intensity is the easier way to have an effect. For example, turning the brightness of your phone down will have a bigger effect than changing the colour. If you do both, it will have an additive effect, but the effect of intensity is much greater than the effect of just colour.
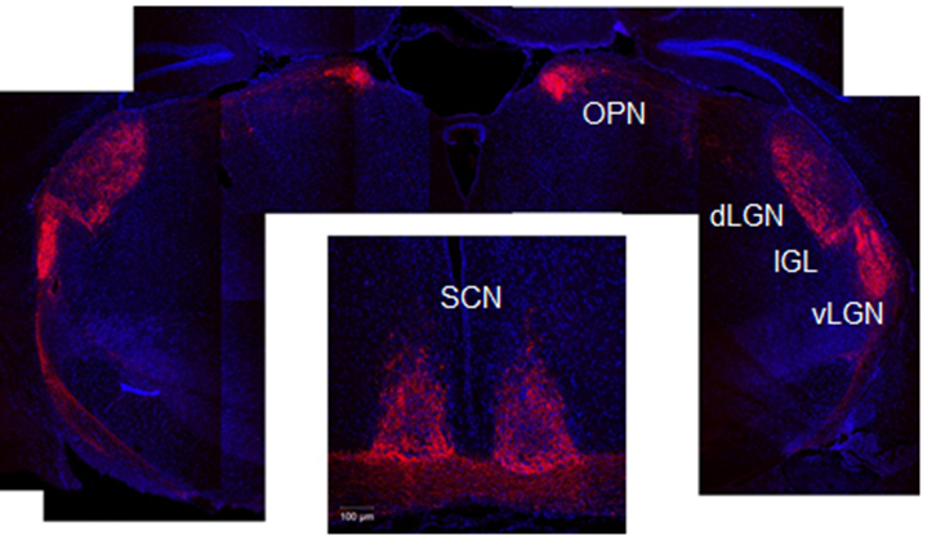
Your recent research has focused on the regulation of circadian rhythms and sleep in mammals. How does decision-making in mice affect their light exposure and what have been your key findings when you recreated the intensity and colour of daylight in lab conditions and when you used intensity ramps to simulate dawn and dusk?
One of the key things we’ve been doing is giving animals the ability to regulate their light exposure. This was an obvious thing to do as we don’t see mice very often during the day as they live in burrows and are more active at night. As a burrowing species, their choice of when to emerge alters their light exposure. And so, our work has been giving animals the option of avoiding light, which leads to them spending less than an hour a day in the light.
We observe more naturalistic behaviour as the animals emerge and start sampling their light environment around dawn and dusk. And when we give a ramped dawn and dusk, we start seeing big spikes as the animals put their heads out of the shelter to judge the light based on the colour and environmental light levels to decide when they’re going to emerge.
Circadian clocks are important in a burrowing species because they don’t know what’s happening outside and so having that internal representation of time is important to know when to emerge.
Colour is important for the circadian clock. The way we detect colour in the retina is the relative activation of two cone channels in a mouse and three with red, green, and blue in the case of a human. The colour that animals see when they look out of their nest box also gives them time information, which shows that the melanopsin system is not working by itself.
In terms of extrapolating this to humans, it is not just changing the light in our environment that may be important, but behavioural modifications such as going outside getting light and our choice of light environment. Our decision-making affects our light exposure, but this behavioural component isn’t often factored in particularly well.
Do the building recommendations cover the colour of light as well as intensity?
A lot of what is happening now in terms of the lighting recommendations has used the integration of melanopsin information, but that doesn’t take the colour factor into account. A lot of building design is related to photopic lux in the environment, which is a measurement of visual brightness to the human retina.
Interestingly, this brightness perception is based on the psychophysical measurements of a handful of human subjects, measured by visual psychophysicists here at UCL. Candela, which is the SI base unit, is based upon that human brightness perception.
Amazingly, despite only being based on a handful of people, this works! There are people who have differences in the visual pigments and have spectral sensitivity, so the lighting unit won’t be quite as accurate for them. But this comes back to my point about blue light. While it is not going to be exactly accurate, it is accurate enough, as the intensity has a much bigger effect apart from when we’re under very controlled lab conditions.
Red light is commonly thought to be invisible to rodents, but recent research has brought this assumption into question. What are your thoughts on red light in mice and how does this impact the circadian rhythm?
The way we look at responses to light is by generating dose-response curves. We change the light intensity and then we look at the biological response with that changing light intensity. If you look at visual response, or circadian response, or any other behavioural response in mice, you will see a characteristic shape of the dose-response curve where you go from no response, to an increasing response, to when it saturates.
Looking at published data from the last 30 years, you can see that it is possible to get a saturating dose response curve in a mouse shifting its circadian clock with red light, you just need a lot more of it – i.e. mice are less sensitive to red light – not blind to it. And so the critical point is the intensity of the light.
At low light levels, red light will not be detectable by mice effectively. This is because rats and mice are less sensitive to red light ~600 nm. Mice are about twelve times less sensitive than a human, but if you make that light twelve times brighter, they will see it just the same. So it is all about relative sensitivity. Humans can even see infrared light if it is really bright!
What advice do you have for mouse husbandry based on your research?
The guideline paper we published earlier this year was a result of a collaboration between people working on mouse vision, mouse circadian rhythms, laboratory animal welfare including people from the RSPCA and animal facility managers. Our main recommendation is that we should be measuring light not just in the room, but what the animals actually experience in the cage.
The animal’s experience matters. For example, animals at the top of the rack may have a 15-fold higher light intensity to those at the bottom. We also recommend measuring light intensity from the animal’s perspective in units that actually relate to mouse biology. For example, it is possible to measure in melanopic lux for mice.
Our more recent research shows the importance of giving animals the opportunities to escape light. Because if mice are under a 12/12 light/dark cycle, given the option, they will typically spend very little time in the light – less than 1 hour per day.
What is the next piece of the puzzle you hope to solve?
In addition to methods development, we are doing a lot of work on recreating the effects of dim light in the evening on animal models. We’re also working on the sleep and circadian rhythm disruption that occurs in mental health conditions. We recently started a new project with a number of collaborators across Oxford going from animal models up to using things like health informatics data from UK Biobank, all the way up to patient interventions where you’re looking to improve sleep using digital cognitive behavioural therapy for insomnia and new pharmacological agents.
There seems to be a strong association between depression, anxiety, psychosis and sleep and circadian rhythm disruption. And improving sleep in patients improves can improve the disease relevant symptoms.
We want to understand why the two are linked because at the moment we have no mechanistic understanding of that. One of the things that we’re starting to look at it is functional brain networks using resting state fMRI going from mouse to human.
We also have a side project on magnetoreception, which is the ability to detect magnetic fields. The reason we’re interested in this area is because the protein that’s been suggested to detect magnetic fields in animals is supposed to be expressed in the retina. The protein is called cryptochrome, which is actually part of the circadian clock.
Birds are thought to use cryptochrome to detect magnetic fields for migration. But we’ve also got some data showing that mice may be able to detect magnetic fields as well, so what cryptochrome may be doing is modulating visual sensitivity.
Does your work have any potential implications for insomnia and jet lag in humans?
We published some work on the molecular mechanism of jet lag a few years ago. Certainly, there seems to be a limit to how quickly our circadian clock can shift – so it is usually about a day for every hour. If you fly across six time zones, it will take six days for your body to catch up.
Our work showed the molecular reason for that limit on the phase shifting is that when you shift the molecular mechanism of the clock, you change the expression of some of the clock genes within the SCN, the master clock in the hypothalamus. But rather than it just keeping on changing, there’s negative feedback on that light input. And so, as the clock starts to shift, the mechanism switches off, so it stops shifting.
If you block this mechanism, you can catch up on the time zone much more quickly. The issue however is that this clock mechanism occurs in cells and tissues throughout the body, so you may not want to start messing up the clock as it could have side effects throughout the body.
Also there are behavioural interventions that you can do instead. For example, you can start shifting your light exposure and mealtimes a bit before you travel and then when you switch to the new time zone, you have started that shifting process already.
This is very important for athletes who have to travel across multiple time zones and play competitive matches. Those travelling are at a disadvantage. This has been shown in work done in the US with basketball and American football players, where the team that travels across more time zones doesn’t perform as well.
In addition to shifting your light exposure and mealtimes, you can also consider taking things like melatonin to help start shifting as well. This is giving your body the cue of the time zone you want to be on before you actually get there. Getting exposure to light at your new destination is also helpful.
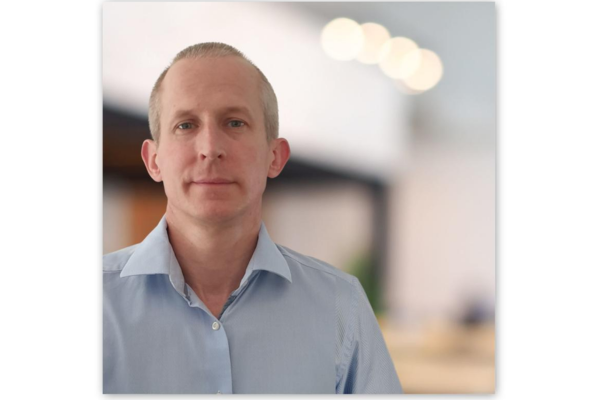
About Professor Stuart Peirson
Stuart Peirson is Professor of Circadian Neuroscience and Deputy Director of the Sleep and Circadian Neuroscience Institute (SCNi) at the University of Oxford. After a degree in Neuroscience at Sheffield University, he studied for a PhD at UCL, then worked as a postdoc and lecturer at Imperial College, before moving to Oxford in 2006. The Peirson Lab is primarily interested in how light regulates physiology and behaviour. As well as vision, the retina contains photoreceptors that regulate many different processes, such as circadian rhythms, sleep and cognitive function. The central aim of his work is to understand how light information is transmitted from photoreceptor to brain to drive these responses. The group has a particular interest in how our modern artificial light environment affects health, and in the mechanisms underlying the disruption of sleep and circadian rhythms in mental health disorders. Professor Peirson was part of the team that first characterised these circadian photoreceptors in humans, as well as leading research on the molecular mechanisms of jetlag and circadian disruption in mouse disease models. He was also a key contributor to lighting guidelines for circadian responses that have now been adopted by the International Lighting Committee (CIE) and are widely used in building design. In addition to serving on multiple animal ethics committees, he chairs the University of Oxford’s 3Rs sub-committee and has developed many novel non-invasive methods for studying mouse physiology and behaviour.